You’ll find that ecology explores how living things interact with each other and their environment. It’s a fascinating field that reveals nature’s complex relationships, from tiny organisms to entire ecosystems. You’ll discover how energy flows from the sun through food chains, how species adapt to survive, and how nutrients cycle through the environment. The science spans multiple levels of organization, from individual cells to the entire biosphere, showing how everything connects. As you explore these intricate ecological relationships, you’ll uncover the delicate balance that sustains life on Earth and shapes our natural world.
What Is Ecology
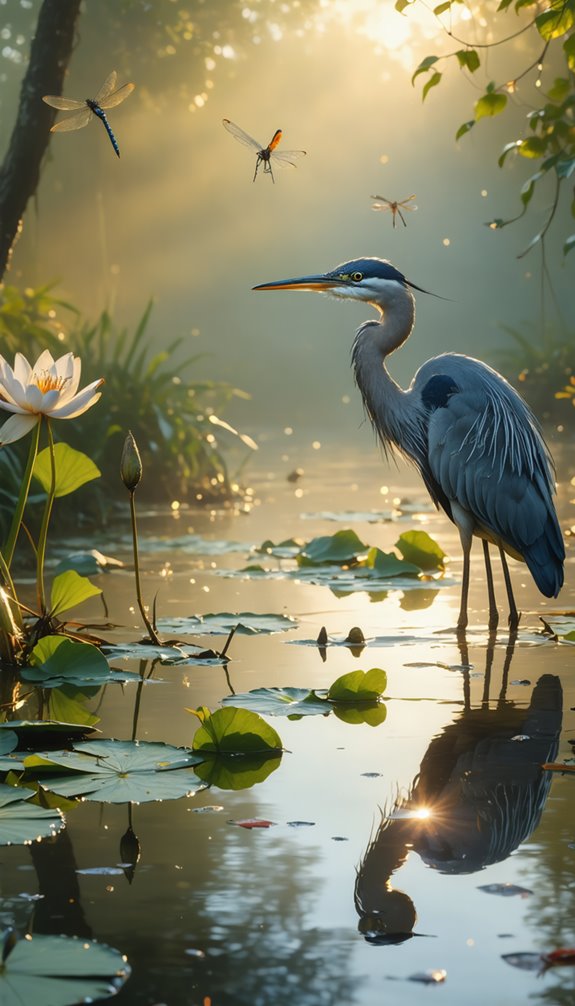
Ecology explores how living things interact with each other and their environment. When you study ecology, you’ll discover how organisms adapt, survive, and thrive within their habitats. You’re examining complex relationships between species, including predator-prey dynamics, competition for resources, and mutually beneficial partnerships.
As an ecological observer, you’ll notice patterns across different scales. At the individual level, you’re watching how a single organism responds to environmental conditions. Moving up to populations, you’re analyzing groups of the same species living together. Communities show you how different species coexist and influence each other. At the ecosystem level, you’re seeing how living and non-living components work together.
You’ll find that ecology connects various biological concepts. When you investigate food webs, you’re seeing energy flow through systems. If you examine plant growth, you’re studying nutrient cycles. By observing migration patterns, you’re learning about climate’s impact on behavior. Ecology helps you understand Earth’s biodiversity and explains why protecting natural systems is essential for all life forms’ survival.
Basic Ecological Concepts
You’ll need to understand two key building blocks of ecology: populations, which are groups of the same species living together, and communities, which are different populations interacting in the same area. As you study these ecological relationships, you’ll see how organisms form complex networks where each species affects others through competition, predation, and cooperation. These interactions create pathways for energy to flow through ecosystems, typically starting with the sun’s energy being captured by plants and moving through various organisms in food chains and food webs.
Population and Communities
Living organisms rarely exist in isolation – they form populations of their own species and interact with other species in communities. When you study populations, you’ll find groups of individuals from the same species living together in a defined area. These populations grow, shrink, and adapt based on factors like food availability, predation, and environmental conditions.
Communities take this concept further, as they’re made up of different populations living and interacting in the same area. You’ll see these interactions everywhere – from birds eating insects to plants competing for sunlight. These relationships can be beneficial (mutualism), harmful (parasitism), or neutral (commensalism).
Within communities, you’ll observe fascinating dynamics like competition, predation, and symbiosis. Species often compete for limited resources such as food, water, or territory. Predator-prey relationships help regulate population sizes, while symbiotic relationships show how species can depend on each other for survival. You’ll notice that changes in one population can create ripple effects throughout the entire community, demonstrating the interconnected nature of ecological systems.
Energy Flow Through Ecosystems
Through ecosystems, energy flows in a one-way direction, starting with the sun and moving through different organisms. You’ll find that producers, mainly green plants, capture this solar energy through photosynthesis and convert it into chemical energy stored in glucose. When consumers eat these producers, they’re accessing this stored energy.
You can trace energy as it moves through trophic levels, from primary consumers (herbivores) to secondary consumers (carnivores) and sometimes to tertiary consumers (top predators). At each level, you’ll notice that only about 10% of the energy transfers to the next level, while 90% is lost as heat or used for the organism’s life processes.
When organisms die or produce waste, decomposers break down this organic matter, releasing nutrients back into the ecosystem. While matter cycles within an ecosystem, energy doesn’t – it’s continuously flowing through the system and eventually dissipates as heat. This is why ecosystems need a constant input of solar energy to maintain their function and why you’ll always find producers at the base of every energy pyramid.
Levels of Ecological Organization
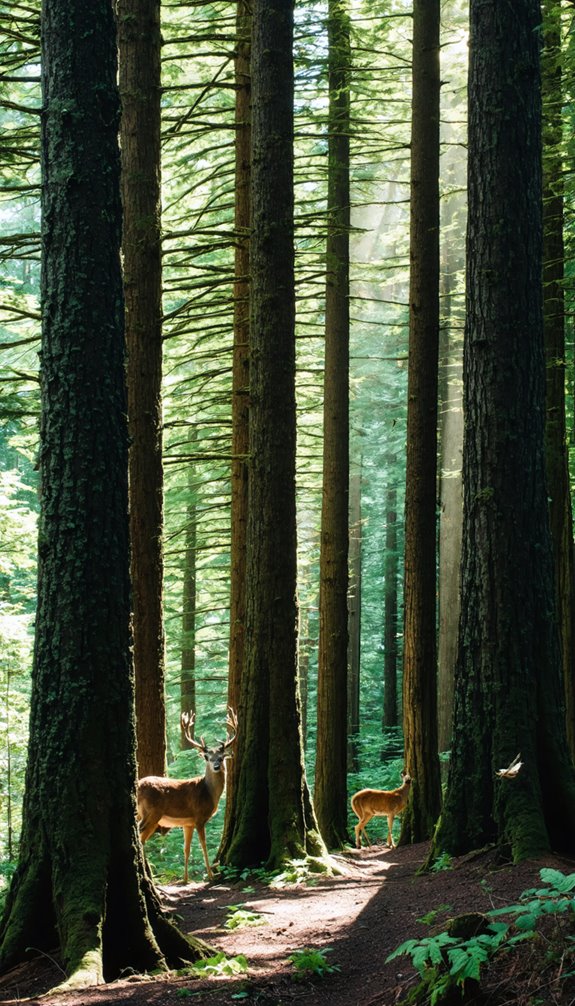
You’ll find that ecology spans multiple levels of organization, from the tiniest atoms up through cells, organisms, and ultimately to the entire biosphere that encompasses Earth. Within this hierarchy, you can observe how populations of species form communities that interact through competition, predation, and cooperation. As matter and energy flow between these organizational levels, you’ll see how each component plays an essential role in maintaining the balance of ecosystems.
From Atoms to Biosphere
Everything in ecology follows a hierarchical structure, from the smallest components of life to Earth’s entire living system. You’ll find that this organization starts with atoms, which combine to form molecules, which then create the organelles and cells that make up living organisms. These cells work together to form tissues, and tissues combine to create organs that function as part of larger organ systems.
When you look at the bigger picture, you’ll see that individual organisms of the same species form populations. These populations don’t exist in isolation – they interact with other species’ populations in communities. These communities, along with their physical environment, create ecosystems like forests, lakes, or coral reefs. As you expand your view further, you’ll notice that similar ecosystems grouped together form biomes, such as tropical rainforests or tundra. Finally, at the highest level, all of Earth’s biomes, together with their organisms and physical environments, form the biosphere – our planet’s entire living system. Each level builds upon the previous one, creating increasingly complex ecological relationships.
Populations and Communities Connect
Populations and communities stand out as two essential interconnected levels in ecological organization. When you study populations, you’ll find groups of organisms from the same species living and interacting in a specific area. These populations don’t exist in isolation – they’re part of larger, more complex systems called communities, where different species coexist and interact.
You’ll notice that populations connect with communities through various relationships. Within a forest community, for instance, you can observe how a population of oak trees provides food and shelter for populations of squirrels and birds. These interactions include predator-prey relationships, competition for resources, and mutually beneficial partnerships called symbiosis.
Understanding these connections helps you grasp how changes in one population can ripple through an entire community. If a disease affects a population of bees, you’ll see impacts on plant populations that depend on them for pollination. This, in turn, affects herbivores that feed on those plants, demonstrating how population dynamics influence community structure and stability. You can’t fully understand one level without considering its relationship to the other.
Energy Flow Between Levels
Energy flows through ecological levels in a fascinating and predictable pattern, starting with the sun’s radiation and moving through producers, consumers, and decomposers. You’ll find that plants and other autotrophs capture only about 1% of available sunlight, converting it to chemical energy through photosynthesis. These producers then become food for primary consumers, which are eaten by secondary consumers, creating a chain of energy transfer.
At each level, you’ll notice that only about 10% of the energy transfers to the next trophic level, while 90% is lost as heat or unused material. That’s why you won’t find more than four or five trophic levels in most ecosystems – there simply isn’t enough energy left to sustain additional levels. Decomposers play a vital role by breaking down dead organisms and waste products, recycling nutrients back into the system.
You can visualize this energy flow through ecological pyramids, where the base (producers) contains the most energy and biomass, while each successive level becomes smaller. This hierarchical structure helps you understand why apex predators are relatively rare in nature.
Energy Flow in Ecosystems
The flow of energy through an ecosystem follows a one-way path, unlike the cycling of nutrients. You’ll find that energy enters most ecosystems as sunlight, which plants and other autotrophs capture through photosynthesis. These producers convert solar energy into chemical energy stored in organic compounds, making it available to other organisms.
When you examine food chains, you’ll notice that energy transfers from one trophic level to the next as organisms eat each other. At each transfer, only about 10% of the energy moves to the next level, while 90% is lost as heat or used for the organism’s life processes. That’s why you’ll typically find fewer organisms at higher trophic levels.
You can observe this energy flow through various feeding relationships. Primary consumers eat producers, secondary consumers eat primary consumers, and tertiary consumers eat secondary consumers. Decomposers break down dead organisms and waste products, releasing energy as heat. This process demonstrates why energy must constantly enter the ecosystem through sunlight, as it can’t be recycled like nutrients can.
Food Chains and Webs
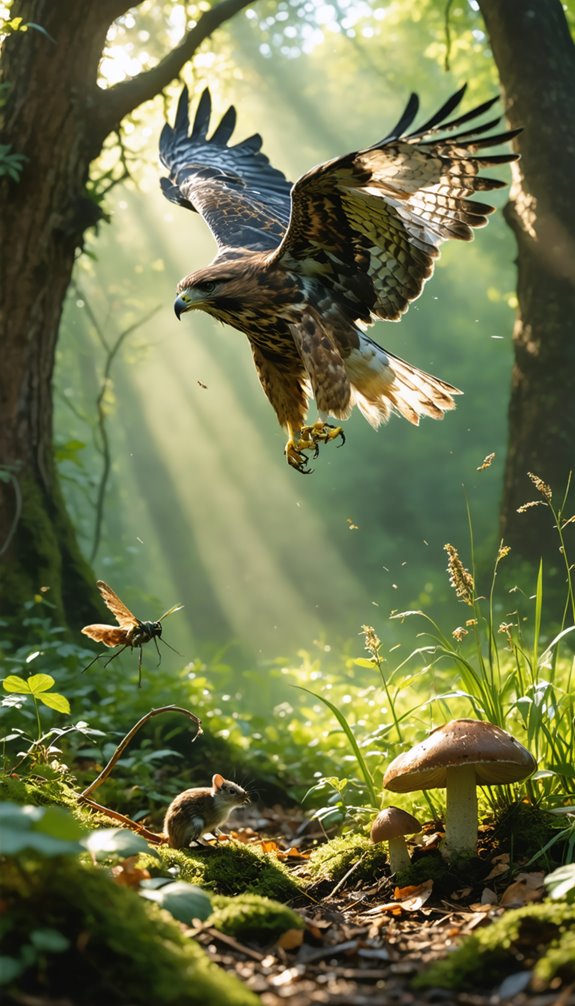
Living organisms regularly interact through feeding relationships that form both simple food chains and complex food webs. You’ll find that food chains represent a linear sequence of who eats whom, starting with producers like plants that convert sunlight into energy through photosynthesis. These producers are eaten by primary consumers (herbivores), which are then consumed by secondary consumers (carnivores), and sometimes by tertiary consumers (top predators).
In nature, you’ll rarely see simple food chains because most organisms eat multiple species and are eaten by various predators. That’s where food webs come in – they’re interconnected networks of food chains that show all the feeding relationships in an ecosystem. You can think of food webs as a more realistic representation of how energy flows through an ecosystem. When you examine a food web, you’ll notice that some species, called keystone species, play essential roles in maintaining the web’s stability. If you remove these keystone species, you’ll often see dramatic changes in the ecosystem’s structure and function. Understanding food webs helps you predict how changes in one population can affect entire communities.
Nutrient Cycling
Nature operates through continuous cycles of essential nutrients like carbon, nitrogen, and phosphorus that move between living organisms and their environment. You’ll find these nutrients continuously recycling through Earth’s ecosystems as they transfer from soil to plants, animals, and back again. When organisms die or produce waste, decomposers break down the organic matter, releasing these nutrients back into the environment.
The carbon cycle connects all life forms through photosynthesis and respiration. Plants capture carbon dioxide from the air, while animals release it through breathing. You can observe the nitrogen cycle when bacteria convert atmospheric nitrogen into forms that plants can use, which then moves up the food chain. The phosphorus cycle primarily occurs between land and water, as this vital nutrient weathers from rocks and cycles through living systems.
These biogeochemical cycles don’t operate in isolation – they’re interconnected and depend on both biological and geological processes. When you understand nutrient cycling, you’ll see how disruptions in one cycle can affect others, impacting entire ecosystems. That’s why maintaining these natural cycles is significant for Earth’s ecological balance.
Population Dynamics
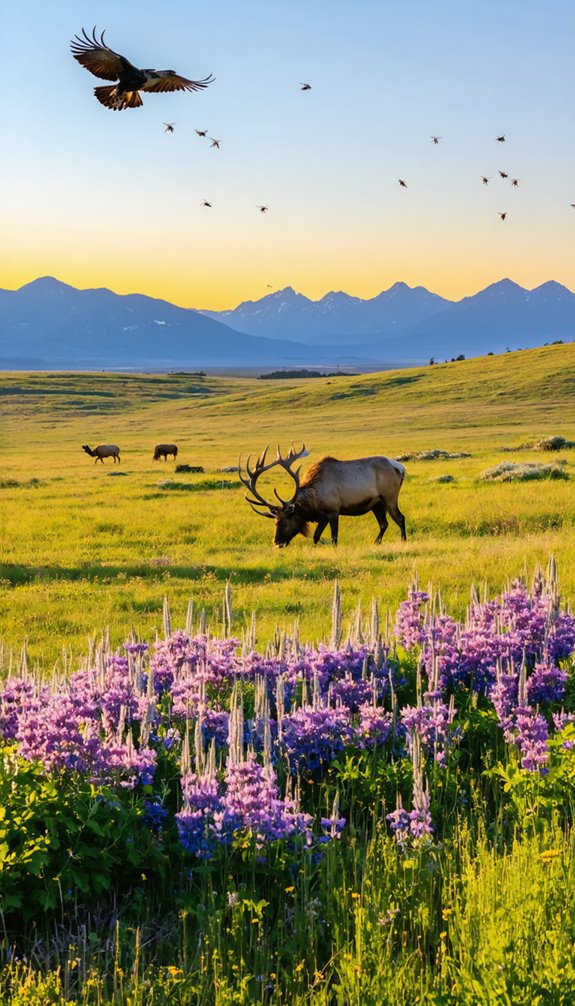
You’ll find that population dynamics center on how groups of organisms change over time through growth and mortality patterns. When you study species interactions, you’ll notice that competition plays a vital role in determining which populations thrive and which decline. Your understanding of these patterns helps explain how different species survive in their ecosystems, adapting to both environmental pressures and the presence of other organisms.
Growth and Mortality Patterns
Populations exhibit distinct patterns of growth and mortality that shape their overall dynamics in ecosystems. You’ll find that population growth typically follows one of two main patterns: exponential or logistic growth. In exponential growth, you’ll see numbers increase rapidly when resources are abundant, while logistic growth shows an S-shaped curve as populations approach their carrying capacity.
When you’re studying mortality patterns, you’ll notice they’re often age-dependent. Type I mortality curves show low death rates until late in life, which you’ll commonly see in humans and large mammals. Type II curves display constant mortality rates throughout life, as you’d observe in many birds. Type III curves reveal high mortality in early life stages, which you’ll find in most fish and invertebrates.
You can’t separate growth from mortality, as they’re intrinsically linked. Environmental factors like predation, disease, and resource availability will affect both patterns simultaneously. You’ll need to take into account density-dependent factors, which become more intense as population size increases, and density-independent factors, such as natural disasters, which affect populations regardless of their size.
Species Competition and Survival
When organisms share limited resources in an ecosystem, competition becomes a driving force that shapes their survival and distribution. You’ll find that species compete for essential resources like food, water, territory, and mates. This competition occurs both within species (intraspecific) and between different species (interspecific), leading to various survival strategies and adaptations.
In your study of competition, you’ll notice that species often develop specialized traits to reduce competition pressure. Some organisms might adjust their feeding times, utilize different parts of shared resources, or adapt to unique niches. You’ll see this in action when birds of different species feed at various heights in the same tree, or when plants develop different root depths to access water and nutrients.
Competition’s intensity depends on resource availability and population density. When resources are scarce, you’ll observe stronger competitive interactions, which can result in the exclusion of weaker competitors. This process helps maintain ecosystem balance and drives evolutionary changes, as species must either adapt to new conditions or risk local extinction.
Species Interactions
Throughout nature, species constantly interact with each other in ways that shape their survival, behavior, and evolution. You’ll find five main types of species interactions: competition, predation, parasitism, mutualism, and commensalism. Each of these relationships plays a vital role in maintaining ecosystem balance.
When you observe predation, you’re seeing one species (the predator) hunting and consuming another (the prey). In parasitism, one organism benefits while harming its host, like when ticks feed on mammals. Competition occurs when species fight for limited resources, such as birds competing for nesting sites or plants struggling for sunlight.
You’ll notice that not all interactions are harmful. In mutualism, both species benefit – think of bees pollinating flowers while collecting nectar. Commensalism is where one species benefits while the other isn’t affected, like small fish using sharks for protection without impacting the shark. Understanding these interactions helps you grasp how ecosystems function and evolve. They’re not static relationships but dynamic connections that can change over time as species adapt to environmental pressures.
Habitat and Niche
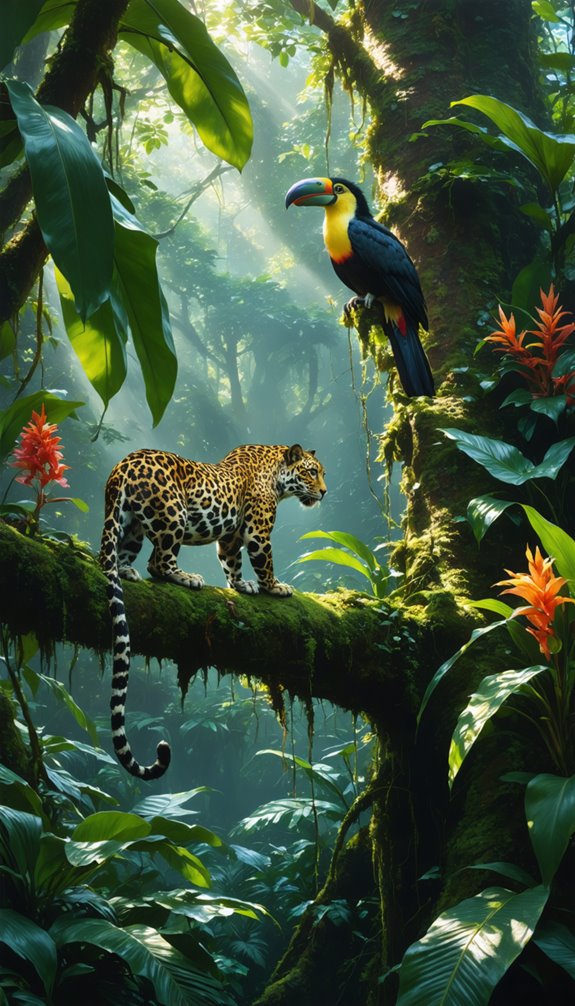
When you study habitat and niche, you’ll find that while a habitat is the physical location where an organism lives, a niche represents its complete ecological role including its interactions, behaviors, and resource requirements. You’ll discover three main types of ecological niches: fundamental niches (where species could potentially live), realized niches (where they actually live), and hypothetical niches (predicted spaces species might occupy). Resource partitioning occurs when species share a habitat but avoid competition by using different resources, operating at different times, or occupying distinct spaces within that environment.
Defining Habitat Vs Niche
The distinction between habitat and niche represents one of ecology‘s most fundamental concepts. While you’ll often hear these terms used interchangeably, they describe very different aspects of how organisms interact with their environment. Think of habitat as an organism’s physical address – the actual location where it lives and can be found. For example, a penguin’s habitat includes the Antarctic coastline and surrounding waters.
A niche, however, encompasses much more than just location. It’s the organism’s complete role in its ecosystem, including what it eats, when it’s active, how it behaves, and how it interacts with other species. You can think of a niche as an organism’s occupation or lifestyle within its habitat. A penguin’s niche includes being a daytime predator that feeds on fish and krill, nests in colonies, and serves as prey for killer whales and leopard seals. The same habitat can support multiple species, but each typically occupies a distinct niche to avoid direct competition. This concept, known as niche differentiation, helps explain how different species can coexist in the same space while utilizing different resources.
Types of Ecological Niches
Within ecological systems, scientists recognize three main types of niches: fundamental, realized, and potential. You’ll find that a fundamental niche represents the entire range of conditions under which an organism could potentially survive and reproduce if there weren’t any competitors or limiting factors. It’s basically the organism’s theoretical maximum capacity for existence.
The realized niche is what you’ll actually observe in nature – it’s smaller than the fundamental niche because it accounts for biological interactions like competition, predation, and disease. For example, a species might theoretically be able to live in a broader temperature range, but competitors restrict it to a smaller area.
A potential niche describes conditions where an organism could potentially thrive but hasn’t yet colonized. You’ll see this concept when studying invasive species – they might discover and exploit potential niches in new environments. When you’re analyzing how species adapt to environmental changes, you’ll notice that their realized niches can shift between these different types, especially when facing competition, climate change, or other environmental pressures.
Resource Partitioning Between Species
Resource partitioning represents nature’s solution to competitive exclusion, where species living in the same area divide available resources to minimize direct competition. You’ll find this ecological concept at work when different species share the same habitat but utilize resources in distinct ways to avoid conflict.
You can observe resource partitioning through various mechanisms. Species might feed at different times of day, like nocturnal owls and diurnal hawks hunting the same prey. They may use different parts of the same resource, such as giraffes feeding on upper tree branches while antelope graze on lower vegetation. Some species partition space by occupying different vertical layers of the habitat – think of birds nesting at different heights in a forest canopy.
When you study resource partitioning, you’ll notice it often involves differences in physical characteristics. A classic example is Darwin’s finches, where beak sizes evolved to exploit different food sources. These adaptations don’t just happen by chance – they’re the result of long-term evolutionary processes that help species reduce competition and coexist successfully in the same ecosystem.
Biodiversity and Species Richness
Biodiversity encompasses three distinct levels of life’s variety: genetic diversity within species, species richness across communities, and ecosystem diversity across landscapes. You’ll find that species richness, the total number of different species in a given area, serves as a key indicator of an ecosystem’s health and resilience. When you measure biodiversity, you’re actually quantifying the complexity of life’s web in that location.
You can assess biodiversity using various metrics, including the Simpson’s diversity index and Shannon-Wiener index, which consider both species richness and evenness. These measurements help you understand how species are distributed within an ecosystem. For instance, you’ll see higher biodiversity scores in areas where species are more evenly represented, compared to areas dominated by just a few species.
You’ll notice that biodiversity often increases as you move from poles to tropics, and it’s typically higher in areas with stable climates, abundant resources, and minimal disturbance. Understanding these patterns helps you predict how environmental changes might affect species distributions and ecosystem stability, making biodiversity monitoring essential for conservation efforts.
Ecosystem Services
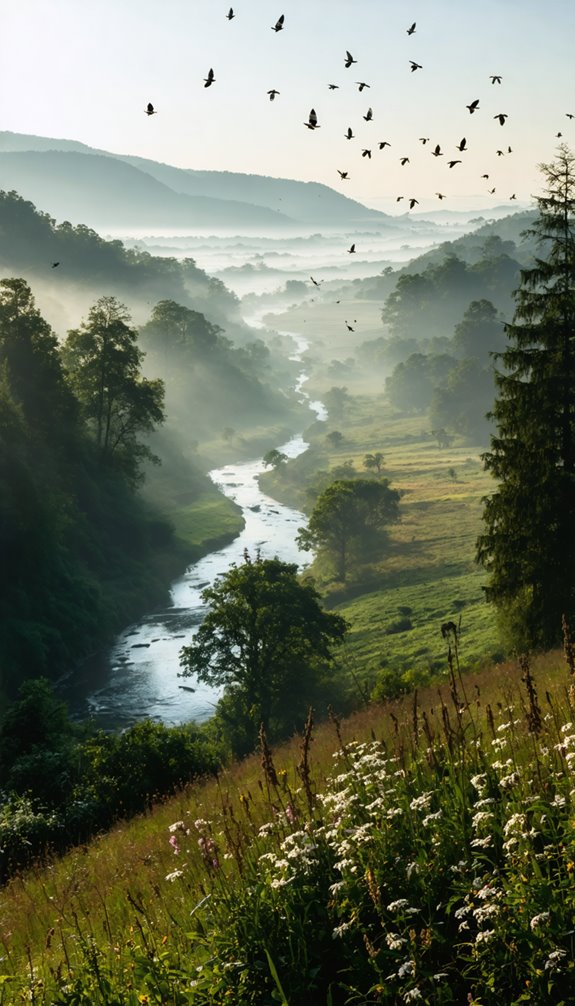
You’ll find that ecosystem services are crucial for your survival, providing everything from clean air and water to food production and climate regulation. Your well-being directly depends on these natural processes that work together to maintain Earth’s life-support systems. By protecting these crucial services, you’re safeguarding the natural capital that sustains both human societies and global ecosystems.
Benefits for Human Survival
Our survival as a species depends heavily on the countless benefits that natural ecosystems provide, known as ecosystem services. You’ll find these services essential for your daily life, from the air you breathe to the food you eat. Forests and oceans absorb carbon dioxide and produce oxygen, while soil microorganisms break down organic matter to create fertile ground for agriculture.
You rely on natural pollinators like bees and butterflies to maintain food production, as they’re responsible for pollinating roughly 35% of global crops. When you drink clean water, you’re benefiting from nature’s filtration systems – wetlands, soil layers, and aquatic organisms that purify water naturally. You’ll also find protection from natural disasters through ecosystem services: mangroves and coral reefs buffer coastal areas from storms, while forests prevent soil erosion and regulate water flow to reduce flooding.
Even your medicine cabinet contains numerous drugs derived from natural compounds found in plants, fungi, and marine organisms. These ecosystem services aren’t just convenient – they’re irreplaceable and crucial for your continued existence on Earth.
Natural Capital Protection
Through careful management and protection of natural capital, societies can guarantee the long-term sustainability of essential ecosystem services. You’ll find that natural capital encompasses Earth’s natural assets, including soil, air, water, and all living organisms, which work together to provide humans with significant resources and services.
When you protect natural capital, you’re safeguarding important ecosystem services like water purification, carbon sequestration, and pollination. You can see this in action when wetlands filter pollutants from water, forests absorb carbon dioxide, and bees pollinate crops. By maintaining these natural systems, you’re ensuring they’ll continue providing services worth trillions of dollars annually.
To protect natural capital effectively, you’ll need to implement various strategies. These include establishing protected areas, adopting sustainable resource management practices, and reducing pollution. You can also support natural capital by investing in restoration projects, implementing green infrastructure, and developing policies that recognize the economic value of ecosystem services. Remember that every action you take to protect natural capital helps secure these significant services for future generations.
Natural Selection
According to Charles Darwin’s groundbreaking theory, natural selection is the process that drives evolution, where organisms with beneficial traits survive and reproduce more successfully than others. You’ll find that these advantageous traits are passed down to offspring, gradually becoming more common in populations over generations. Natural selection operates through several key mechanisms: variation, inheritance, competition, and differential survival.
When you observe natural selection in action, you’ll notice it’s not a conscious process but rather an automatic result of environmental pressures. For instance, if you’re studying a population of moths, those with coloring that better matches their surroundings will be more likely to avoid predators and survive to reproduce. Over time, you’ll see this protective coloration become more prevalent in the population.
You can identify four main types of natural selection: stabilizing, directional, disruptive, and sexual selection. Each type affects trait distribution differently within populations. For example, when you’re examining directional selection, you’ll notice it pushes a population toward one extreme of a trait’s range, like longer beaks in birds that feed on specific foods.
Adaptation and Evolution
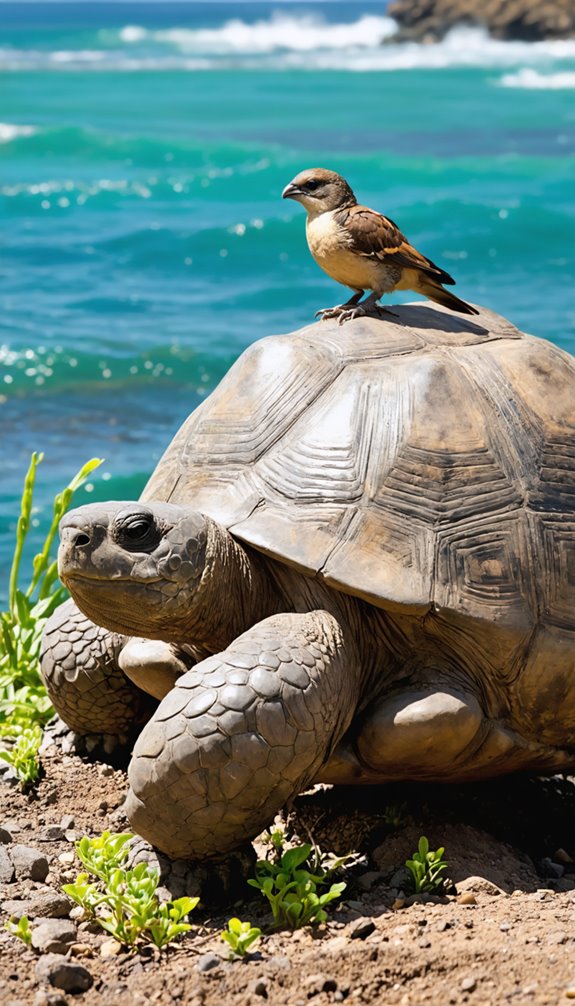
You’ll find that natural selection shapes species through inherited traits that improve survival and reproduction rates. Environmental pressures, such as extreme temperatures, limited food sources, and predator threats, force organisms to adapt or face extinction. These pressures create evolutionary changes over time, as you can observe in examples like the Arctic fox’s white fur or the cactus’s water-storing capabilities.
Natural Selection Fundamentals
Nature’s most powerful mechanism for change, natural selection, drives the remarkable process of evolution through successive adaptations. You’ll find that natural selection occurs when organisms with favorable traits survive and reproduce more successfully than others in their population. These advantageous characteristics get passed down to offspring, becoming more prevalent in subsequent generations.
To understand natural selection, you need to recognize its four key components. First, there’s variation – individuals in a population possess different traits. Second, these traits must be heritable, meaning they can pass from parents to offspring. Third, organisms produce more offspring than can survive, leading to competition. Fourth, some variations provide advantages in survival and reproduction.
You’ll see natural selection in action when you observe examples like antibiotic-resistant bacteria or pesticide-resistant insects. These populations change because individuals with resistance genes survive and reproduce, while others die off. The process isn’t random; it’s a direct response to environmental pressures. When you combine this mechanism with time, you’ll understand how species can transform dramatically, adapting to their changing environments.
Environmental Pressure Examples
Three powerful examples demonstrate how environmental pressures drive adaptation and evolution in the natural world. You’ll find the first example in the peppered moth of England, where industrial pollution during the 1800s blackened tree bark with soot. Light-colored moths became easy targets for predators, while darker moths survived and passed on their genes, shifting the population’s color within decades.
You can observe the second example in Africa’s cichlid fish, where different water depths and food sources have led to diverse jaw shapes and feeding mechanisms. In shallow waters, you’ll find cichlids with strong jaws for crushing snails, while in deeper waters, cichlids have developed specialized jaws for catching prey.
The third example lies in the Galápagos finches, where you’ll notice thirteen distinct species evolved from a single ancestor. Each species has developed unique beak shapes adapted to specific food sources – from cracking nuts to probing flowers. During droughts, you can witness natural selection in action as finches with better-suited beaks survive at higher rates, demonstrating evolution’s response to environmental challenges.
Climate and Weather
Throughout Earth’s history, climate and weather have shaped the development of life and ecosystems in profound ways. You’ll find that climate represents long-term patterns of temperature, precipitation, and atmospheric conditions in a region, while weather describes day-to-day variations in these same elements. When you study ecology, you’ll need to understand how both factors influence species distribution and adaptation.
You can observe climate’s impact in the way it determines which organisms can survive in specific regions. For instance, you’ll see cacti thriving in hot, dry deserts, while moss flourishes in cool, damp forests. Weather events, like storms, droughts, and cold snaps, create immediate challenges that organisms must overcome to survive.
If you’re examining an ecosystem, you’ll notice that climate and weather affect everything from breeding seasons to migration patterns. They influence when plants flower, when insects emerge, and when birds migrate. You’ll also find that these factors determine soil moisture, water availability, and nutrient cycling – all essential elements that support life in any ecosystem.
Biomes of Earth
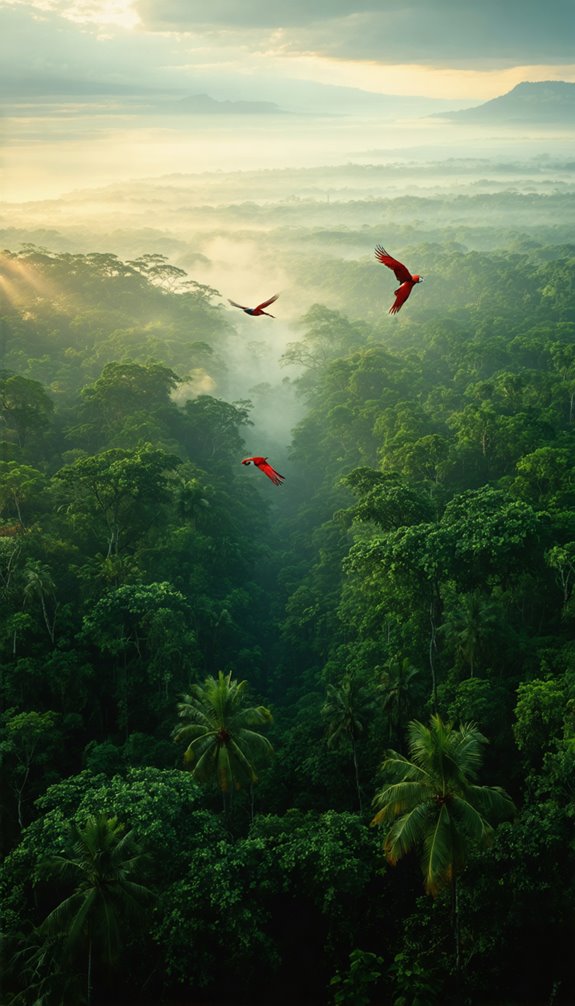
Major biomes spread out across Earth’s surface in distinct patterns, each shaped by the interplay of temperature, precipitation, and latitude. You’ll find tropical rainforests near the equator, where warm temperatures and abundant rainfall create perfect conditions for diverse plant and animal life. As you move away from the equator, you’ll encounter savannas with their scattered trees and extensive grasslands.
In temperate zones, you’ll see deciduous forests that showcase dramatic seasonal changes, while coniferous forests dominate higher latitudes with their cold-resistant evergreen trees. Desert biomes exist where rainfall is scarce, creating harsh conditions where specialized organisms thrive. Tundra regions occupy the planet’s northernmost areas, characterized by permafrost and limited vegetation.
You can also observe how elevation affects biome distribution. As you climb mountains, you’ll notice distinct vegetation zones that mirror latitude changes – from tropical forests at the base to alpine tundra at the peak. Each biome supports unique communities of organisms that have adapted to its specific environmental conditions, creating Earth’s remarkable biodiversity.
Aquatic Ecosystems
You’ll find aquatic ecosystems are broadly categorized into marine and freshwater habitats, each supporting unique food chains from microscopic phytoplankton to apex predators. Within freshwater systems, you’ll encounter three main types: lakes, streams, and wetlands, which provide essential breeding grounds and resources for diverse species. As you explore coastal regions, you’ll notice specialized adaptations that allow organisms to thrive in the challenging intersection of land and sea, where salinity, tides, and wave action shape the ecosystem’s characteristics.
Marine Food Chain Dynamics
Marine food chains form the backbone of Earth’s aquatic ecosystems, connecting diverse organisms through intricate feeding relationships. You’ll find these chains begin with microscopic phytoplankton, which harness sunlight through photosynthesis to produce energy. These tiny producers support countless zooplankton species, creating the foundation for all marine life above them.
As you examine the next levels, you’ll discover small fish feeding on zooplankton, while larger predatory fish consume these smaller species. The chain continues upward to apex predators like sharks, orcas, and tuna. You’ll notice that energy transfer between each level isn’t completely efficient – only about 10% of energy moves from one trophic level to the next.
You can observe how these marine food chains interweave to form complex food webs, where species often occupy multiple trophic levels. When you study these relationships, you’ll see how disruptions at any level can trigger cascading effects throughout the ecosystem. Changes in phytoplankton populations, for instance, can impact fish stocks, seabird populations, and even top predators, demonstrating the delicate balance that maintains marine biodiversity.
Freshwater Habitat Types
While ocean ecosystems cover vast expanses of our planet, freshwater habitats represent distinct aquatic environments that support unique forms of life. You’ll find these habitats in three main categories: lentic (standing water), lotic (flowing water), and wetlands.
In lentic systems, you’ll encounter lakes and ponds where water movement is minimal. These bodies of water form distinct layers based on temperature and light penetration, creating various ecological niches from the surface to the bottom. You’ll notice that deeper lakes often have three zones: the littoral, limnetic, and profundal.
Lotic systems include rivers and streams where you’ll observe constant water movement. These habitats create unique adaptations in organisms that must resist current flow. You’ll find different communities in rapids versus pools, each adapted to specific flow conditions.
Wetlands represent areas where water and land intersect. You’ll discover marshes, swamps, and bogs in these conversion zones. They’re essential for flood control and water filtration, and you’ll find specially adapted vegetation that can thrive in saturated soils.
Coastal Ecosystem Adaptations
Coastal habitats present three distinct challenges that shape how organisms adapt: constant wave action, tidal fluctuations, and salinity changes. You’ll notice that organisms in these environments have developed specialized features to survive these harsh conditions. To combat wave action, many species like barnacles and mussels have strong adhesive substances and hard shells, while seaweeds possess flexible stems that bend rather than break.
During tidal cycles, you’ll find that organisms must cope with alternating periods of submersion and exposure. Many intertidal species can seal themselves off from the environment – barnacles close their shell plates, while sea anemones retract their tentacles and contract their bodies. Some creatures, like fiddler crabs, synchronize their activities with tidal rhythms.
When it comes to salinity fluctuations, you’ll observe that coastal organisms have evolved sophisticated mechanisms. Salt marsh plants can excrete excess salt through specialized glands, while many fish and invertebrates use osmotic regulation to maintain their internal salt balance. Some organisms, like mangroves, have developed specialized root systems that filter out salt as they take in water.
Terrestrial Ecosystems
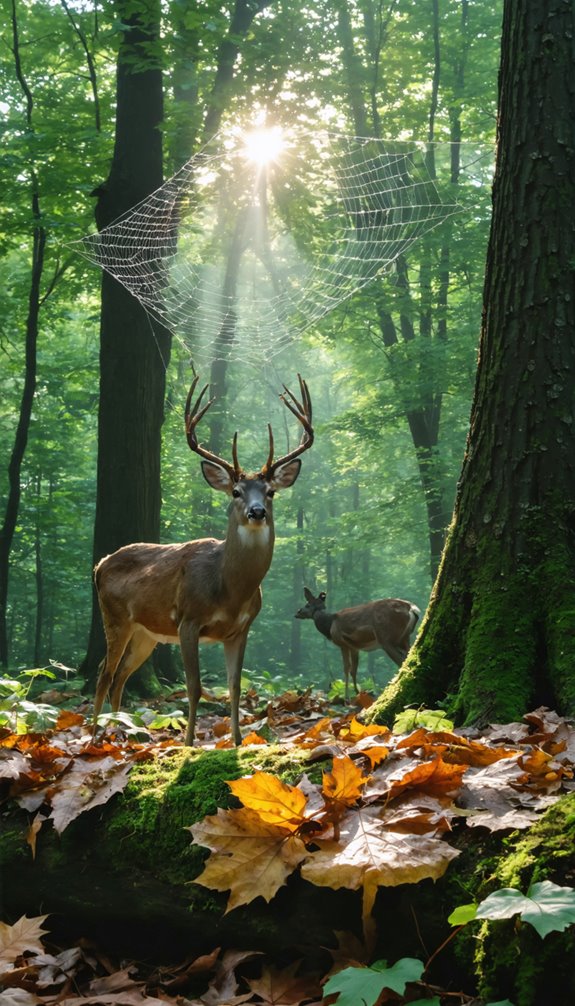
Terrestrial ecosystems cover roughly 30% of Earth’s surface and include diverse environments from dense rainforests to arid deserts. You’ll find these ecosystems characterized by their soil types, climate conditions, and the organisms that have adapted to live within them. From the towering sequoias of temperate forests to the hardy cacti of deserts, each species plays a vital role in maintaining ecological balance.
When you examine terrestrial ecosystems, you’ll notice they’re organized into distinct biomes based on temperature, rainfall, and latitude. The tundra’s frozen grounds support specialized plants and animals adapted to extreme cold, while tropical rainforests burst with biodiversity due to consistent warmth and rainfall. You’ll discover that grasslands act as vast carbon sinks, while deciduous forests showcase seasonal adaptations through leaf loss and regrowth.
What makes these ecosystems particularly fascinating is how they’re interconnected through nutrient cycles and energy flow. You can observe how decomposers break down organic matter, herbivores regulate plant populations, and predators maintain species balance. Understanding these relationships is essential for conservation efforts and predicting ecosystem responses to environmental changes.
Urban Ecology
Modern cities represent unique ecosystems where human infrastructure intersects with natural processes. You’ll find that urban environments create distinct microclimates, with temperatures typically 2-8°F warmer than surrounding rural areas due to the heat island effect. This phenomenon occurs when buildings and pavement absorb and retain heat, affecting both wildlife behavior and plant growth patterns.
Within cities, you’ll observe remarkable adaptations as species adjust to human presence. Birds like pigeons and sparrows have learned to nest in building facades, while raccoons and coyotes have modified their hunting strategies to take advantage of human refuse. You can spot urban plants growing in unexpected places, from wall cracks to rooftop gardens, demonstrating nature’s resilience.
When you study urban ecology, you’ll notice how cities function as novel ecosystems with their own food webs and nutrient cycles. Human activities directly influence these systems through pollution, waste management, and landscape modification. Understanding these interactions helps you develop sustainable urban planning strategies that support both human needs and ecological processes, making cities more livable for all species.
Conservation Biology
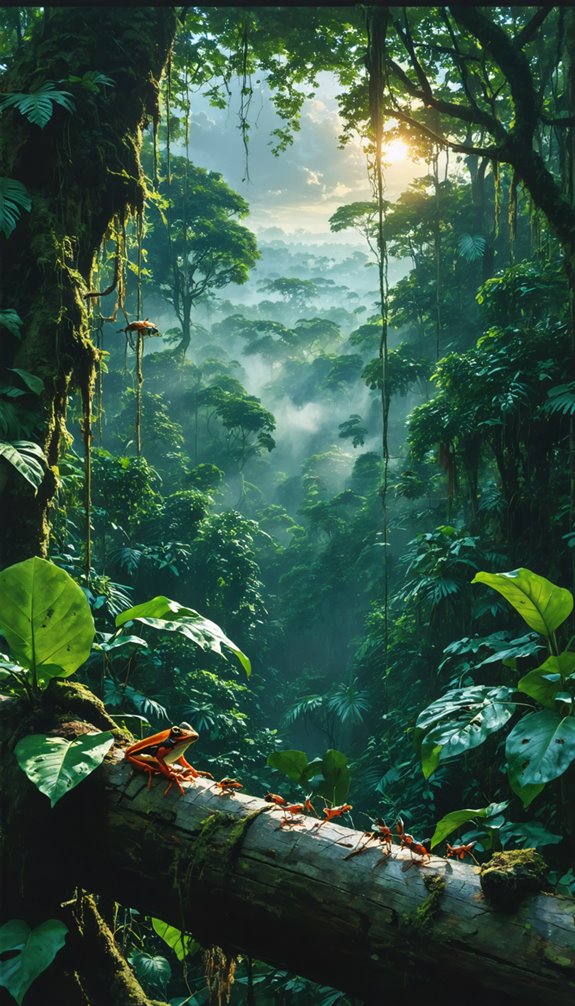
Conservation biology steps up to address one of humanity’s most pressing challenges: preventing species extinction and preserving Earth’s biodiversity. You’ll find this field combines ecological principles with practical strategies to protect endangered species and their habitats. When you study conservation biology, you’re learning how to identify threatened populations, assess their viability, and develop effective management plans.
To protect biodiversity, you must understand both in-situ and ex-situ conservation methods. In-situ conservation lets you preserve species in their natural habitats through protected areas, wildlife corridors, and habitat restoration. Ex-situ conservation involves maintaining species outside their natural environments, like in zoos, botanical gardens, and seed banks.
You’ll need to evaluate genetic diversity, minimum viable populations, and metapopulation dynamics when developing conservation strategies. Conservation biologists also tackle issues like habitat fragmentation, invasive species, and climate change impacts. By working with local communities, policymakers, and other stakeholders, you’ll help implement sustainable solutions that balance human needs with biodiversity protection. The field’s success depends on your ability to integrate scientific knowledge with practical conservation actions.
Environmental Stressors
You’ll encounter three main categories of environmental stressors that affect organisms and ecosystems: physical factors like temperature and light, chemical impacts from pollutants and pH changes, and biological pressures from competition and predation. These stressors don’t work in isolation but often interact with each other to create complex challenges for living organisms. Understanding how these environmental pressures affect species and communities is vital for predicting ecosystem responses and developing effective conservation strategies.
Physical Stress Factors
Living organisms constantly face challenges from their physical environment that can disrupt their normal functioning and survival. You’ll find that temperature acts as one of the most significant physical stressors, as organisms must maintain their body temperature within specific ranges to survive. When it’s too hot or too cold, their metabolic processes can slow down or even stop completely.
Water availability is another essential physical stress factor you need to take into account. Organisms can experience both water scarcity and excess water stress, leading to dehydration or drowning. Similarly, light intensity affects photosynthetic organisms dramatically – too much light can damage cellular components, while insufficient light limits energy production.
Physical pressure, whether from altitude changes, ocean depths, or soil compaction, can severely impact organisms. You’ll notice that wind exposure can cause mechanical stress on plants and affect animal behavior. Additionally, organisms must cope with varying levels of oxygen availability, soil or water pH, and salinity. These physical stressors often work in combination, creating complex challenges that require organisms to develop specific adaptations for survival.
Chemical Environmental Impacts
Beyond physical stressors, chemical factors in the environment pose significant challenges to organisms. You’ll find that chemical stressors can be both natural and human-made, affecting ecosystems at multiple levels. These include changes in soil pH, water salinity, toxic metals, pesticides, and atmospheric pollutants.
When you examine natural chemical stressors, you’ll notice that volcanic emissions release sulfur dioxide and other gases, while decomposing organisms produce various chemical compounds that can alter soil composition. You’ll also find that coastal areas experience salt stress from ocean spray, affecting plant growth and soil chemistry.
Human activities have dramatically increased chemical stress factors. You’ll encounter industrial pollutants in urban areas, agricultural runoff in farming regions, and acid rain that can impact ecosystems hundreds of miles from its source. These chemicals don’t just affect individual organisms – they create ripple effects throughout food webs. For instance, when you study mercury contamination, you’ll see how it biomagnifies up the food chain, becoming more concentrated in top predators. Understanding these chemical impacts helps you predict and manage ecological responses to environmental change.
Biological Pressure Effects
Throughout ecosystems, biological pressures create complex webs of stress that shape survival and adaptation. You’ll find these pressures manifesting through competition, predation, parasitism, and disease – all of which force organisms to evolve or perish. When you examine predator-prey relationships, you’ll notice how they’ve led to the development of camouflage, defensive structures, and escape mechanisms.
Competition for resources adds another layer of biological pressure. You can observe this when plants compete for sunlight, water, and nutrients, leading to specialized root systems and varying leaf arrangements. In animal populations, you’ll see competition drive territorial behavior, mating strategies, and feeding patterns.
Population density creates its own set of pressures. When you study overcrowded populations, you’ll find increased disease transmission, reduced reproductive success, and heightened aggression. These density-dependent factors often trigger behavioral changes and population control mechanisms. You can also witness how invasive species introduce new biological pressures, disrupting established ecological relationships and forcing native species to adapt or decline. Understanding these biological pressures helps you predict ecosystem changes and implement effective conservation strategies.
Ecological Succession
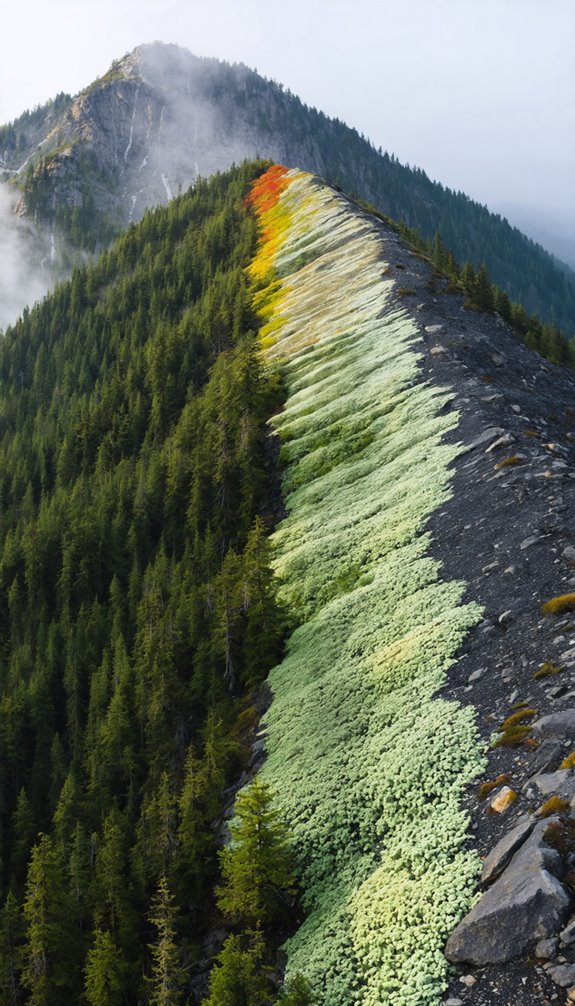
In natural environments, communities of organisms undergo predictable changes over time through a process called ecological succession. You’ll find two main types: primary succession, which begins in lifeless areas like bare rock or newly formed volcanic islands, and secondary succession, which occurs in areas where an ecosystem previously existed but was disturbed.
During primary succession, you’ll observe pioneer species like lichens and mosses first colonizing bare substrate. These organisms break down rocks and begin forming soil, making way for larger plants. In secondary succession, you’ll see faster changes because soil already exists. After a forest fire, for example, you’ll notice grasses and weeds appearing first, followed by shrubs and small trees.
As succession progresses, you’re watching an ecosystem become more complex. Early colonizers change the environment, creating conditions that allow other species to thrive. The process continues until the ecosystem reaches a relatively stable state called climax community. However, you should understand that external factors like climate change, natural disasters, or human activities can reset or alter this progression at any time.
Keystone Species
Ecosystems frequently depend on certain essential organisms called keystone species, which have an outsized impact on their environment compared to their relative abundance. You’ll find that these species play a significant role in maintaining ecological balance, even though they might represent a small portion of the ecosystem’s biomass.
When you study keystone species, you’ll discover classic examples like sea otters in kelp forests. They control sea urchin populations, which would otherwise devour kelp beds. Without sea otters, these underwater forests would disappear, affecting countless other species. Similarly, you’ll see how wolves in Yellowstone regulate elk populations, indirectly protecting vegetation and supporting beaver populations.
You can identify keystone species by observing what happens when they’re removed from an ecosystem. Their absence often triggers a cascade of changes that can dramatically alter the entire community structure. For instance, if you remove prairie dogs from grasslands, you’ll notice how the landscape changes as their burrows no longer aerate soil or create habitat for other species. Understanding these relationships helps you predict how ecosystems might respond to environmental changes or conservation efforts.
Invasive Species
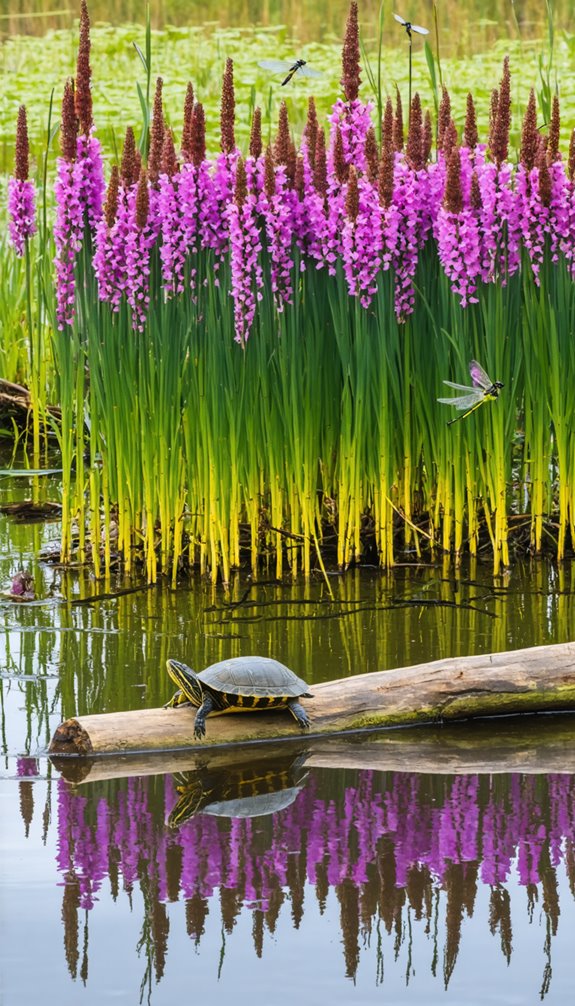
You’ll find that invasive species enter new ecosystems through various pathways, including accidental transport, deliberate introduction, and natural dispersal mechanisms. When you look at their ecological impacts, you’ll see these non-native organisms can dramatically alter food webs, compete with native species, and modify entire habitats. To control and prevent invasive species, you can employ multiple strategies such as biological control agents, physical removal methods, and strict regulatory measures at ports and borders.
Pathways of Species Entry
Invasive species sneak, swim, fly, and crawl into new environments through various pathways, both natural and human-assisted. You’ll find that natural dispersal occurs when organisms move through water currents, wind patterns, or by hitching rides on migratory animals. However, human-mediated pathways have greatly accelerated the spread of invasive species worldwide.
When you’re traveling internationally, you might unknowingly transport invasive species through your luggage, clothing, or shoes. Commercial shipping plays a major role too – cargo vessels carry organisms in ballast water or attached to their hulls. You’ll notice that the pet and ornamental plant trades contribute significantly, as escaped or released species can establish themselves in new areas. Agricultural imports often harbor unwanted hitchhikers like insects or plant diseases.
Construction materials, vehicle movement, and recreational activities can spread invasive species across regions. Even seemingly harmless actions like dumping aquarium contents or moving firewood between locations can create new invasion pathways. By understanding these entry routes, you’ll be better equipped to prevent the spread of harmful invasive species in your community.
Ecological Impact Assessment
Scientists measure the devastating effects of non-native species through ecological impact assessments, which evaluate damage to native biodiversity, ecosystem functions, and economic resources. You’ll find that these assessments track multiple indicators, including population declines of native species, changes in habitat structure, and alterations to essential ecosystem processes like nutrient cycling and pollination.
When you’re examining ecological impacts, you’ll need to reflect on both direct and indirect effects. Direct impacts include predation, competition, and disease transmission, while indirect impacts involve changes to food webs, soil chemistry, and physical habitat modification. For example, when you study the effects of European green crabs, you’ll observe their direct consumption of native shellfish and indirect impacts on seagrass beds through their burrowing activities.
You can quantify these impacts using standardized metrics like decreased species abundance, reduced genetic diversity, and altered ecosystem services. The assessment data you collect helps prioritize management responses and predict future invasion risks, ultimately guiding the allocation of conservation resources where they’re needed most.
Control and Prevention Methods
Controlling and preventing invasive species requires a multi-faceted approach that combines early detection, rapid response, and long-term management strategies. You’ll need to implement regular monitoring programs to identify new invasions early, when they’re easier and less costly to control. This includes using environmental DNA testing, remote sensing, and citizen science initiatives.
Once you’ve detected an invasive species, you must act quickly with appropriate control methods. These can include mechanical removal, such as hand-pulling plants or trapping animals; chemical control using targeted pesticides or herbicides; and biological control through natural predators or competitors. You’ll want to choose methods that minimize impact on native species and ecosystems.
Prevention is your most cost-effective strategy. You can establish quarantine measures, inspect incoming goods and materials, and create buffer zones around protected areas. It’s crucial to understand the pathways through which invasive species enter ecosystems – whether through shipping, agriculture, or pet trade. You’ll also need to educate stakeholders about best practices and regulations to prevent accidental introductions and spread of invasive species.
Predator-Prey Relationships
Three key interactions shape predator-prey relationships in nature: pursuit and capture, defense and evasion, and the ongoing evolution of both species. You’ll find that predators develop specialized hunting techniques, from a cheetah’s speed to an owl’s silent flight, while prey species counter with their own adaptations like camouflage or chemical defenses.
When you study these relationships, you’ll notice they’re cyclical. As predator populations increase, they reduce prey numbers, which then leads to fewer predators due to limited food resources. Once predator numbers drop, prey populations rebound, starting the cycle again. You can observe this pattern in classic examples like the lynx-hare relationship in North America.
These interactions create evolutionary pressure on both species. You’ll see predators becoming more efficient hunters while prey develop better survival strategies. This evolutionary arms race has led to remarkable adaptations: moths that can hear bat echolocation, fish that swim in schools to confuse predators, and plants that produce toxins to deter herbivores. In your ecosystem studies, you’ll discover that these relationships help maintain biodiversity and regulate population sizes.
Competition Among Species
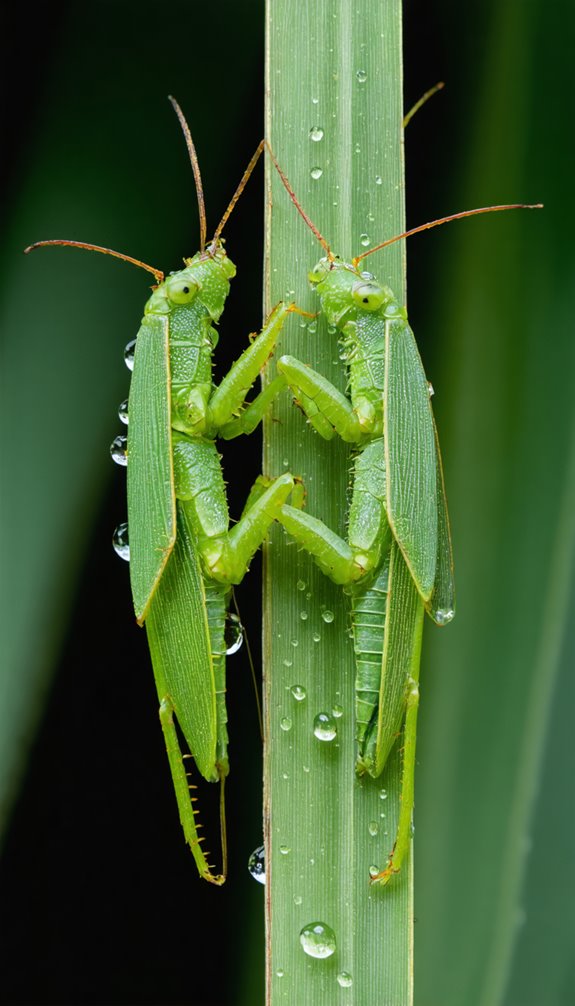
You’ll often see organisms battling for limited resources like food, water, shelter, and breeding grounds in their ecosystems. This fight for survival leads to resource competition, where species using the same resources negatively impact each other’s growth, reproduction, or survival. The competitive exclusion principle states that two species competing for exactly the same resources cannot stably coexist in the same habitat – one will eventually outcompete the other.
Resource Competition Basics
Competition for essential resources shapes the dynamics of ecosystems, as species vie for limited food, water, shelter, and territory. You’ll find that when two or more species require the same resource, they’ll engage in either direct confrontation or indirect competition to secure what they need for survival.
When you examine resource competition, you’ll notice it occurs through two main mechanisms: exploitation and interference. In exploitation competition, species don’t directly interact but deplete the same resource pool. For example, different bird species might feed on the same type of seeds, reducing availability for others. In interference competition, organisms actively prevent others from accessing resources, like when larger trees block sunlight from reaching smaller plants.
You can observe that resource competition intensity varies based on resource abundance and species needs. When resources are scarce, competition becomes more intense, leading to adaptations or niche differentiation. This means species might adjust their behavior, modify their resource use timing, or specialize in using different parts of the same resource to reduce competitive pressure and enable coexistence.
Competitive Exclusion Principle
The competitive exclusion principle states that two species cannot indefinitely occupy the same ecological niche, as one will eventually outcompete and displace the other. You’ll find this principle, also known as Gause’s Law, demonstrated repeatedly in natural ecosystems where species compete for identical resources like food, water, or shelter.
When you observe two similar species coexisting in nature, you’ll notice they’ve actually developed differences in how they use resources. For instance, two bird species might feed on the same type of insect but hunt at different times of day or in different parts of the forest canopy. This resource partitioning helps them avoid direct competition and allows for coexistence.
You can see real-world examples of competitive exclusion in action when invasive species enter new environments. When European starlings were introduced to North America, they outcompeted native cavity-nesting birds for nesting sites. Similarly, when you examine the famous case of Darwin’s finches in the Galápagos Islands, you’ll notice that different species evolved distinct beak shapes to exploit different food sources, preventing competitive exclusion through niche differentiation.
Symbiotic Relationships
In nature, organisms develop close relationships with each other that can last for generations. These interactions between species, called symbiotic relationships, come in three main forms: mutualism, commensalism, and parasitism.
You’ll find mutualism when both species benefit from their relationship. Consider clownfish and sea anemones – the fish gets protection while the anemone receives nutrients from fish waste. In commensalism, one species benefits while the other isn’t affected. You can observe this when cattle egrets follow large mammals, catching insects stirred up by their movement without impacting the mammals.
Parasitism occurs when one organism (the parasite) benefits at the expense of another (the host). You’ll see this in ticks feeding on mammals or tapeworms living in intestines. These relationships can be either endoparasitic (living inside the host) or ectoparasitic (living on the host’s surface).
When you study these relationships, you’ll notice they’re not always fixed – they can shift over time and may vary depending on environmental conditions. Understanding these interactions helps you grasp how species coexist and evolve together in ecosystems.
Ecological Communities
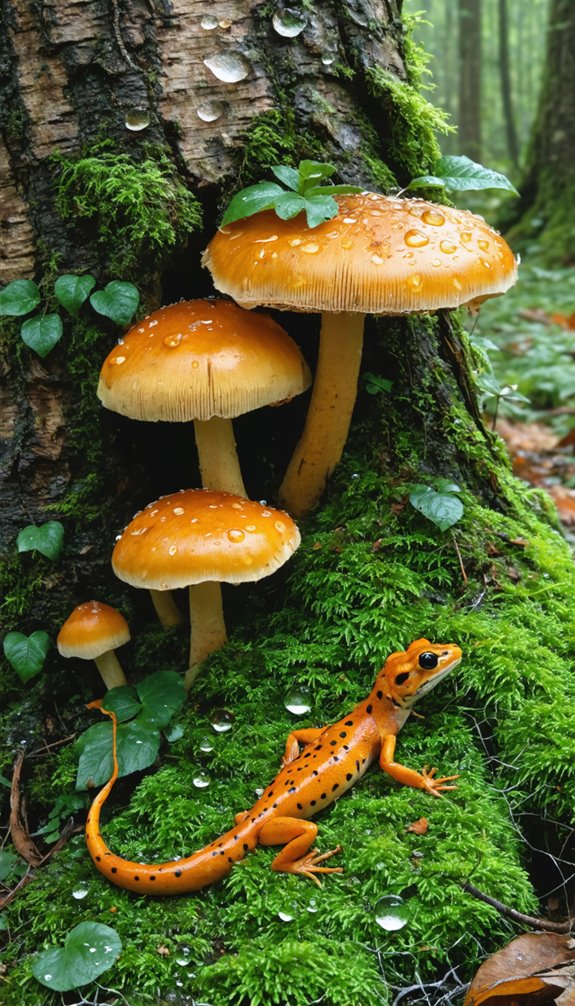
Living organisms never exist in isolation – they form interconnected groups called ecological communities. These communities include all the populations of different species living and interacting within a specific area. When you observe a forest, you’re actually looking at a complex community of trees, shrubs, insects, birds, mammals, fungi, and countless microorganisms.
You’ll find that ecological communities are structured by both biotic and abiotic factors. The species within them compete for resources, prey on each other, and often depend on one another for survival. Weather patterns, soil composition, and available sunlight also shape how these communities develop and maintain themselves.
Within communities, you can identify distinct roles that species play. Some act as producers, creating energy through photosynthesis, while others are consumers at various trophic levels. You’ll notice that each species occupies a specific niche – its unique position and function in the community. Changes to any part of the community can trigger ripple effects throughout the entire system, demonstrating the delicate balance that exists in these natural networks.
Biogeochemical Cycles
You’ll encounter three main biogeochemical cycles when studying how matter moves through Earth’s systems: the carbon cycle that transfers carbon between living things and the atmosphere, the nitrogen cycle that converts nitrogen into usable forms for organisms, and the water cycle that distributes water across the planet. These cycles work together to maintain life and regulate Earth’s climate, with each process connecting living and non-living components in unique ways. While carbon moves primarily through photosynthesis and respiration, nitrogen requires bacterial transformation in soil, and water shifts between solid, liquid, and gas phases as it circulates through the environment.
Carbon Cycle Overview
The carbon cycle stands as Earth’s most critical biogeochemical process, moving this essential element between living organisms, the atmosphere, oceans, and soil. You’ll find carbon everywhere – it’s the backbone of life itself, forming the basis of all organic compounds and playing an essential role in regulating Earth’s climate through atmospheric CO2.
When you look at the cycle’s main components, you’ll see carbon moving through various reservoirs. Plants absorb CO2 during photosynthesis, converting it into glucose and other organic compounds. As animals eat plants, they incorporate this carbon into their tissues. When organisms die or release waste, decomposers break down the organic matter, returning carbon to the soil and atmosphere.
The ocean serves as Earth’s largest carbon sink, where CO2 dissolves into surface waters and gets used by marine organisms. Over millions of years, carbon becomes trapped in sedimentary rocks and fossil fuels. Human activities, particularly burning fossil fuels and deforestation, have greatly altered the natural carbon cycle, leading to increased atmospheric CO2 levels and contributing to global climate change.
Nitrogen Cycle Process
Throughout and across Earth’s biosphere, nitrogen flows in a complex cycle that’s essential for life. You’ll find nitrogen everywhere – it makes up 78% of Earth’s atmosphere, but most organisms can’t use it in its atmospheric form (N₂). That’s where nitrogen-fixing bacteria come in, converting N₂ into usable compounds through a process called nitrogen fixation.
When you look at soil, you’ll see nitrogen fixation happening in two ways: free-living bacteria convert N₂ directly, while symbiotic bacteria work within plant root nodules. The bacteria transform nitrogen into ammonium (NH₄⁺), which plants can absorb. Other bacteria convert ammonium to nitrites (NO₂⁻) and then to nitrates (NO₃⁻) through nitrification. You’ll notice plants readily absorb these nitrates through their roots.
As organisms die or produce waste, decomposers break down organic nitrogen compounds into ammonium in a process called ammonification. The cycle completes when denitrifying bacteria convert nitrates back to N₂, which returns to the atmosphere. You’ll also see this cycle impacted by human activities like fertilizer use and fossil fuel combustion.
Water Cycle Impacts
Water’s constant movement through Earth’s ecosystems drives essential biogeochemical cycles that shape our planet’s climate and sustain life. You’ll find that water cycle impacts extend far beyond simple precipitation and evaporation, as they directly influence soil chemistry, nutrient transport, and atmospheric composition.
When you examine water’s role in weathering rocks, you’ll notice how it dissolves and transports minerals, affecting soil formation and plant nutrition. You can observe this process in action as rainwater carries dissolved nutrients from mountaintops to valleys, enriching ecosystems along the way. The cycle’s disruption can lead to serious consequences – if you’re in an area experiencing drought, you’ll see reduced decomposition rates and slower nutrient cycling.
Water cycle impacts also affect your local climate through evapotranspiration, where plants release water vapor into the atmosphere. You’ll notice this interaction creates microclimates and influences regional weather patterns. Additionally, you’ll find that changes in precipitation patterns can alter ecosystem composition, as species adapt to new moisture conditions or fail to survive in modified environments.
Population Growth
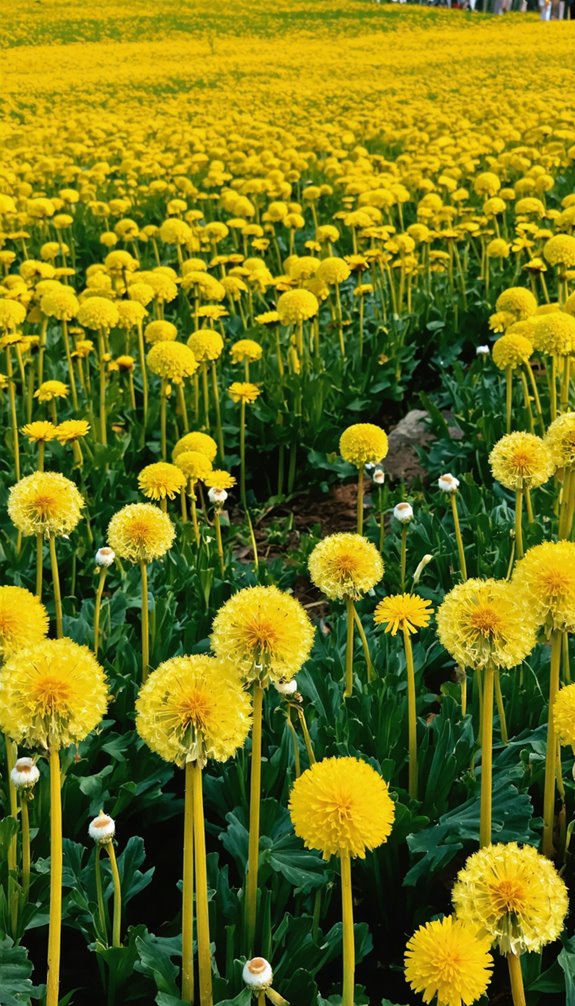
Population growth represents a fundamental concept in ecology, describing how groups of organisms change in size over time. You’ll find that populations can grow exponentially when resources are abundant, doubling at regular intervals as each organism produces offspring. This pattern creates a J-shaped growth curve that you’ll often see in newly introduced species or bacteria in fresh media.
In reality, though, you won’t often observe unlimited growth in nature. As populations increase, they’ll encounter limiting factors like food shortages, predation, or disease. These constraints lead to logistic growth, where you’ll notice the population leveling off at the ecosystem’s carrying capacity. You can identify this pattern by its characteristic S-shaped curve.
You’ll need to take into account both density-dependent and density-independent factors when studying population growth. While competition for resources depends on population size, environmental factors like natural disasters don’t. Understanding these patterns helps you predict how populations will respond to changes in their environment and manage species effectively, whether you’re working in conservation or controlling invasive species.
Carrying Capacity
The concept of carrying capacity defines the maximum population size that an environment can sustainably support over time. When a population reaches this limit, you’ll observe that resource availability, such as food, water, and shelter, becomes a limiting factor for further growth. The environment can’t sustain more individuals without experiencing degradation or collapse.
You can identify when a population approaches its carrying capacity by watching for key indicators. These include increased competition for resources, higher death rates, lower birth rates, and reduced individual fitness. For instance, if you study a deer population in a forest, you’ll notice that as they near carrying capacity, they’ll show signs of malnutrition and decreased reproduction.
It’s important to understand that carrying capacity isn’t static – it changes with environmental conditions. During favorable years, you’ll see it increase due to abundant resources, while during droughts or harsh winters, it’ll decrease. Human activities can also alter carrying capacity by modifying habitats, introducing new species, or changing resource availability. When you’re managing wildlife or studying ecosystems, considering carrying capacity is essential for making informed conservation decisions.
Migration Patterns
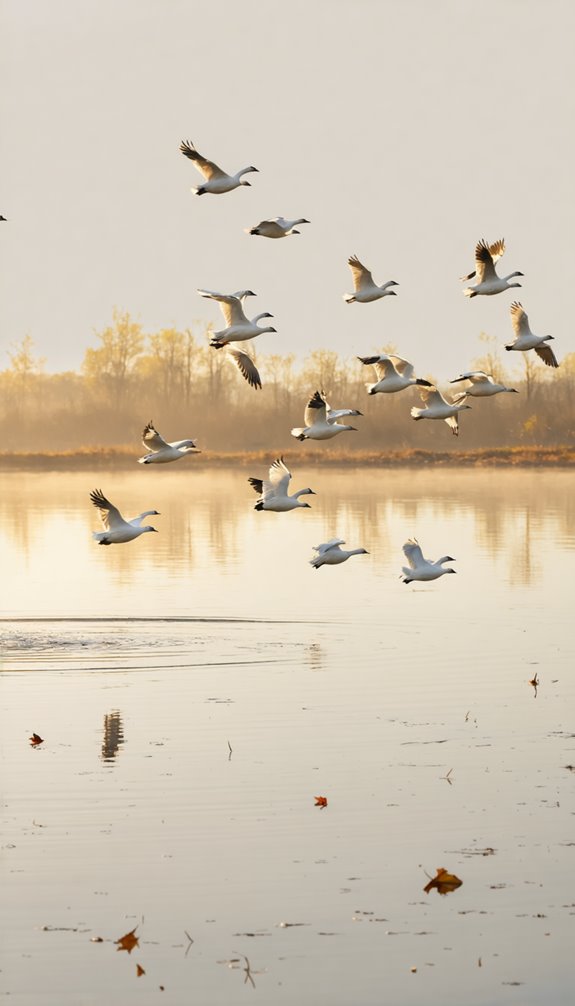
While carrying capacity influences where species can thrive, many animals have evolved remarkable strategies to cope with resource limitations through migration. You’ll find that animals migrate for various reasons: to find food, breed, or escape harsh weather conditions. These journeys can span thousands of miles, like the Arctic tern’s pole-to-pole flight covering 44,000 miles annually.
When you study migration patterns, you’ll notice they’re often cyclical and predictable. Birds follow specific flyways, whales traverse oceanic corridors, and monarch butterflies navigate using the Earth’s magnetic field and solar position. You can observe how these animals time their movements with seasonal changes, following what scientists call “phenological cues.”
You’ll discover that migration isn’t random – it’s a carefully orchestrated process shaped by evolutionary pressures. Animals develop specialized adaptations like fat storage, enhanced navigation abilities, and synchronized breeding cycles. Climate change is now disrupting these age-old patterns, and you can see how some species are adjusting their migration timing or altering their traditional routes in response to shifting temperatures and resource availability.
Behavioral Ecology
Animals constantly make decisions that shape their survival and reproductive success through complex behavioral patterns. As you observe nature, you’ll notice that behavioral ecology explores how these choices evolve and adapt over time to maximize an organism’s fitness. You’ll find that animals develop specific strategies for finding food, avoiding predators, selecting mates, and raising offspring.
When you study behavioral ecology, you’ll see that animals must balance the costs and benefits of their actions. For example, you’ll notice how a bird might risk predator exposure to access better food sources, or how a male peacock’s elaborate tail attracts mates but makes it more vulnerable to predators. You can observe how social behaviors emerge, from the complex hierarchies in wolf packs to the collective decision-making in bee colonies.
You’ll discover that environmental pressures drive these behavioral adaptations. As you examine different species, you’ll find that their behaviors are shaped by factors like resource availability, competition, predation risk, and habitat conditions. Through natural selection, successful behavioral strategies become more common in populations over generations.
Ecological Restoration
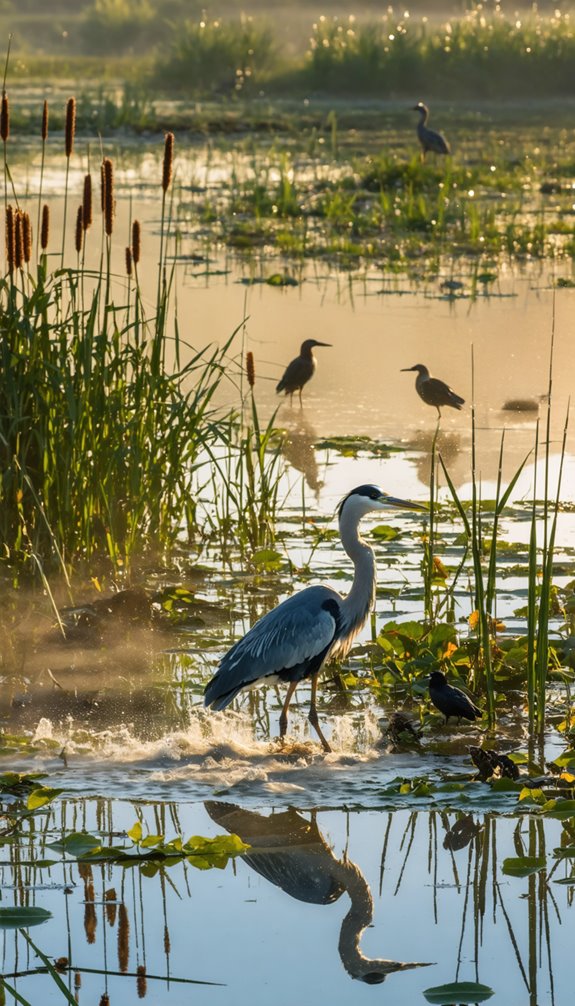
You’ll find that ecological restoration focuses on returning damaged ecosystems to their natural state through carefully planned interventions and management strategies. To achieve this, restoration teams set specific goals like reintroducing native species, removing invasive plants, or improving water quality, then implement methods such as controlled burns, replanting vegetation, or reconstructing wildlife corridors. Success is measured by tracking key indicators like biodiversity levels, soil health, and ecosystem functions compared to reference sites or historical data.
Restoration Goals and Methods
Ecological restoration involves carefully planned interventions to help damaged ecosystems recover their health and functionality. To achieve successful restoration, you’ll need to establish clear goals and select appropriate methods based on your site’s specific conditions. Your primary objectives might include reestablishing native species, improving water quality, enhancing soil health, or restoring natural processes like fire cycles.
You can implement various restoration methods depending on your goals. These include removing invasive species, reintroducing native plants and animals, reconstructing physical habitats, and modifying hydrological conditions. You’ll often need to use multiple techniques in combination. For example, you might first remove invasive plants, then reintroduce native species, and finally establish ongoing management practices to maintain the restored ecosystem.
Before starting any restoration project, you must conduct thorough site assessments and develop detailed plans. You’ll need to monitor progress regularly and adjust your methods as needed. It’s essential to take into account both short-term actions and long-term maintenance strategies to guarantee your restoration efforts create lasting positive changes in the ecosystem.
Measuring Ecosystem Recovery
How do you know if your restoration efforts are actually working? You’ll need to establish specific metrics and monitoring protocols to track ecosystem recovery. You should measure both structural and functional indicators that align with your restoration goals.
Start by collecting baseline data before beginning restoration work. You’ll want to monitor physical parameters like soil composition, water quality, and topographic features. Track biological indicators including species diversity, population sizes, and the presence of key indicator species. Don’t forget to assess ecosystem functions such as nutrient cycling, primary productivity, and habitat connectivity.
You should establish permanent monitoring plots and sampling points for consistent data collection over time. Compare your results against reference sites – healthy ecosystems similar to your target state. You’ll need to monitor both short-term responses (like plant survival rates) and long-term changes (such as community composition). Set specific, measurable targets for each indicator and establish timeframes for achieving them.
Remember that recovery isn’t always linear. You’ll need to practice adaptive management, adjusting your methods based on monitoring results and unexpected challenges that arise during the restoration process.
Human Impact
People have dramatically altered Earth’s ecosystems over the past few centuries through urbanization, industrialization, and population growth. You’ll find these changes manifested in deforestation, habitat fragmentation, and the extinction of numerous species. When you examine human activities closely, you’ll notice they’ve led to widespread pollution of air, water, and soil, while also contributing to climate change through greenhouse gas emissions.
You can observe how agriculture has transformed vast landscapes, with modern farming practices leading to soil degradation and water depletion. If you look at coastal areas, you’ll see how overfishing has disrupted marine ecosystems, while plastic pollution now affects even the most remote ocean regions. Urban development continues to replace natural habitats, and you’ll find that many species can’t adapt to these rapid changes.
As you study human impact, you’ll realize it’s not all negative. You can see successful conservation efforts, sustainable practices, and ecosystem restoration projects worldwide. By understanding how your actions affect the environment, you’re better equipped to make choices that help protect and preserve Earth’s ecological systems for future generations.
Ecological Footprint
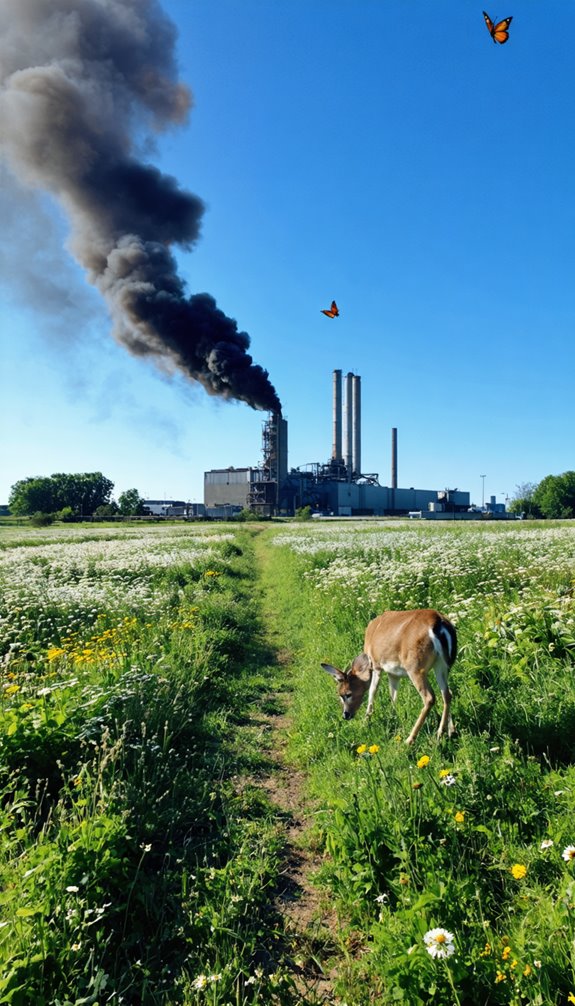
Your ecological footprint measures how your daily activities and consumption habits affect the Earth’s resources. When you look at the bigger picture, global resource consumption shows how humanity’s collective actions strain the planet’s capacity to sustain life. You can reduce your environmental impact through conscious choices like using less energy, minimizing waste, and choosing sustainable products.
Measuring Personal Impact
Through daily activities and lifestyle choices, each individual leaves a measurable mark on Earth’s ecosystems. You can calculate your personal ecological impact by examining key factors like energy consumption, transportation habits, food choices, and waste generation. Your daily commute, household heating and cooling, and electricity usage directly contribute to your carbon footprint.
To measure your impact, you’ll need to track specific metrics: your monthly utility bills, miles driven or flown, meat consumption frequency, and recycling habits. Online calculators can help you convert these numbers into standardized units, typically expressed in global hectares or carbon dioxide equivalents. You can compare your results to national and global averages to understand where you stand.
Understanding your personal impact helps you identify areas for improvement. You might discover that your air travel contributes more to your footprint than your daily driving, or that your meat consumption has a larger impact than your household energy use. By measuring these factors regularly, you can make informed decisions about lifestyle changes that effectively reduce your environmental impact.
Global Resource Consumption
How does humanity’s collective resource consumption impact Earth’s capacity to sustain life? Each year, we’re using more resources than our planet can regenerate, creating an ecological deficit. This overconsumption affects everything from forests and fisheries to freshwater supplies and carbon absorption capacity.
You’ll find that developed nations typically consume resources at a much higher rate than developing countries. For example, if everyone lived like the average American, we’d need about 5 Earths to sustain the global population. This disparity highlights the uneven distribution of resource consumption across the globe.
When you examine global resource usage, you’ll notice several key factors driving overconsumption: population growth, increasing affluence, technological advancement, and changing consumption patterns. These pressures lead to deforestation, soil degradation, water scarcity, and climate change. The challenge we’re facing isn’t just about reducing consumption – it’s about finding ways to meet human needs while staying within Earth’s regenerative capacity. You can contribute to the solution by understanding your consumption patterns and making informed choices about resource use.
Reducing Environmental Impact
Individuals can greatly reduce their environmental impact through simple yet effective lifestyle changes. You’ll find that small adjustments in your daily routine can markedly decrease your ecological footprint. Start by reducing your energy consumption – switch to LED bulbs, unplug unused electronics, and adjust your thermostat settings seasonally.
Your transportation choices matter greatly. Consider walking, cycling, or using public transit when possible. If you must drive, combine multiple errands into single trips and maintain your vehicle properly to optimize fuel efficiency. You can also reduce waste by composting organic materials, recycling consistently, and choosing products with minimal packaging.
Water conservation is essential – install low-flow fixtures, fix leaks promptly, and collect rainwater for garden use. When shopping, opt for local, seasonal produce to reduce transportation emissions and support sustainable agriculture. You’ll make a bigger impact by choosing reusable items over single-use products and by properly maintaining your possessions to extend their lifespan. Consider energy-efficient appliances when replacements are needed, and explore renewable energy options like solar panels for your home.
Sustainable Resource Management
Sustainable resource management forms the backbone of modern ecological practices, ensuring that natural resources remain available for future generations while meeting current needs. You’ll find this approach critical in maintaining the delicate balance between human consumption and nature’s capacity to regenerate.
When you’re implementing sustainable resource management, you’ll need to focus on three key areas: renewable resources, non-renewable resources, and waste management. You can manage renewable resources like forests by ensuring harvest rates don’t exceed regeneration rates. For non-renewable resources such as minerals, you’ll want to invest in recycling programs and alternative materials.
You’ll discover that effective resource management isn’t just about conservation – it’s about optimization. You can achieve this through technologies that improve efficiency, policies that regulate consumption, and practices that minimize waste. By monitoring resource usage patterns and implementing recovery systems, you’re able to create closed-loop systems where waste becomes input for other processes. This approach helps you reduce environmental impact while maintaining economic viability, making it easier to meet both current demands and future sustainability goals.
Wildlife Conservation
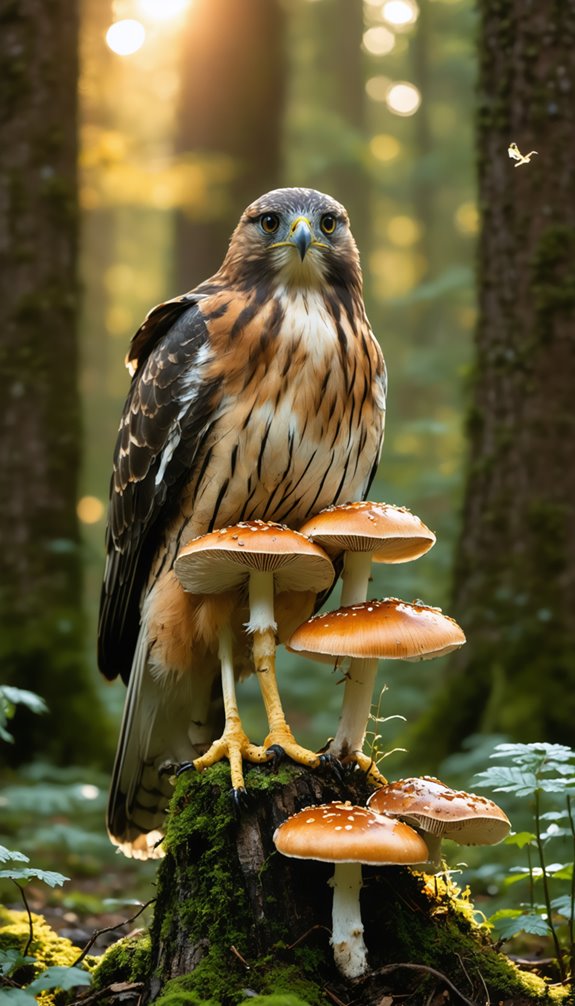
Building on resource management principles, wildlife conservation stands as a cornerstone of ecological preservation. You’ll find that protecting endangered species, maintaining biodiversity, and preserving natural habitats are key elements of this crucial field. When you’re studying wildlife conservation, you’ll need to understand both species-specific needs and broader ecosystem dynamics.
To effectively conserve wildlife, you’ll want to focus on three main strategies. First, you’ll need to protect critical habitats through the establishment of reserves and national parks. Second, you must address human-wildlife conflicts by implementing buffer zones and developing community-based conservation programs. Third, you’ll need to support species recovery through breeding programs and population monitoring.
You can participate in wildlife conservation through various means. Whether you’re joining local conservation groups, supporting anti-poaching initiatives, or contributing to habitat restoration projects, your actions make a difference. Remember that successful wildlife conservation depends on scientific research, public education, and international cooperation. By understanding these principles, you’re better equipped to contribute to the preservation of Earth’s biodiversity.
Habitat Fragmentation
Devastating effects of habitat fragmentation pose one of the most significant threats to global biodiversity. When you observe a landscape that’s been divided by roads, urban development, or agriculture, you’re witnessing habitat fragmentation in action. This process breaks continuous habitats into smaller, isolated patches, making it difficult for species to maintain viable populations.
You’ll find that fragmentation creates several challenges for wildlife. Edge effects increase as the ratio of edge to interior habitat grows, exposing more animals to predation and environmental stresses. Species that require large territories can’t survive in small habitat patches, and populations become isolated, leading to reduced genetic diversity and increased inbreeding.
If you’re studying fragmented landscapes, you’ll notice that many species can’t move between habitat patches, especially when the matrix (the area between fragments) is hostile to wildlife movement. This isolation prevents animals from accessing resources, finding mates, or adapting to environmental changes. Wildlife corridors can help mitigate these effects by connecting isolated patches, but they don’t fully compensate for the loss of continuous habitat.
Global Climate Change
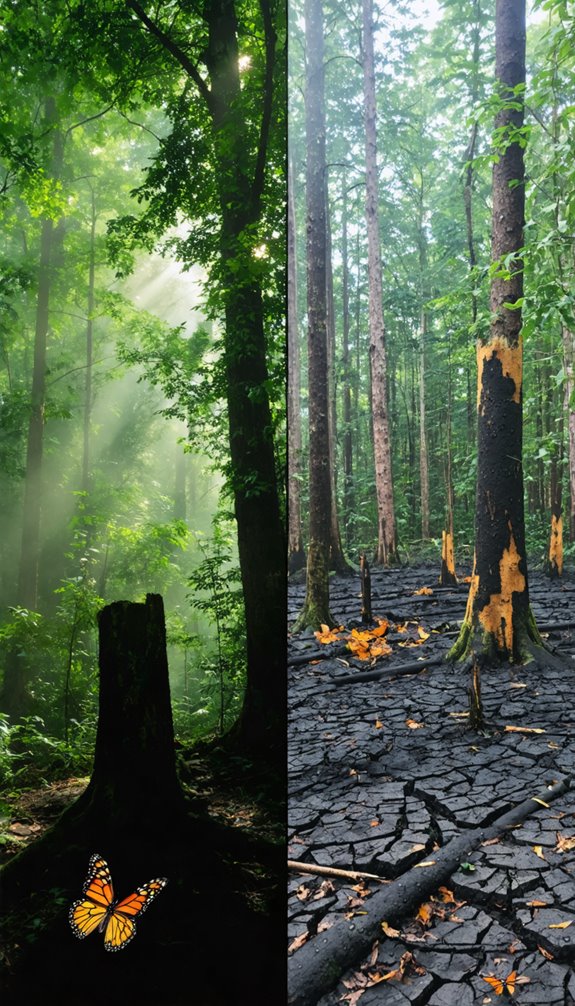
Earth’s climate system faces unprecedented changes as greenhouse gas emissions continue to trap heat in the atmosphere. You’ll notice these changes through rising global temperatures, melting polar ice caps, and more frequent extreme weather events. The primary drivers are human activities like burning fossil fuels, deforestation, and industrial processes that release carbon dioxide, methane, and other greenhouse gases.
You can observe climate change’s impacts on ecosystems worldwide. Species are shifting their ranges toward the poles or to higher elevations as their habitats warm. Many plants and animals can’t adapt quickly enough to these rapid changes, leading to increased extinction risks. You’ll find that seasonal timing of biological events, like flowering and migration, has shifted, disrupting ecological relationships that evolved over millennia.
If you examine ocean ecosystems, you’ll see additional effects. Rising sea temperatures are causing coral bleaching, while ocean acidification threatens marine organisms that build calcium carbonate shells. These changes ripple through food webs, affecting everything from plankton to top predators, fundamentally altering marine ecosystem dynamics.
Endangered Species
While countless species naturally go extinct over time, human activities have accelerated extinction rates to unprecedented levels. You’ll find that human-caused threats like habitat destruction, pollution, climate change, and overexploitation have pushed many species to the brink of extinction. Scientists estimate that current extinction rates are 100 to 1,000 times higher than natural background rates.
When you examine endangered species, you’ll notice they’re classified based on their risk of extinction. The International Union for Conservation of Nature (IUCN) maintains the Red List, which categorizes species from “least concern” to “extinct.” You can identify critically endangered species like the black rhino, mountain gorilla, and hawksbill sea turtle, which face imminent threats to their survival.
Conservation efforts you might encounter include protected areas, breeding programs, and international treaties like CITES (Convention on International Trade in Endangered Species). You’ll find that protecting endangered species isn’t just about preserving biodiversity – it’s essential for maintaining ecosystem stability, as each species plays a significant role in its environment’s complex web of interactions.
Ecological Methods
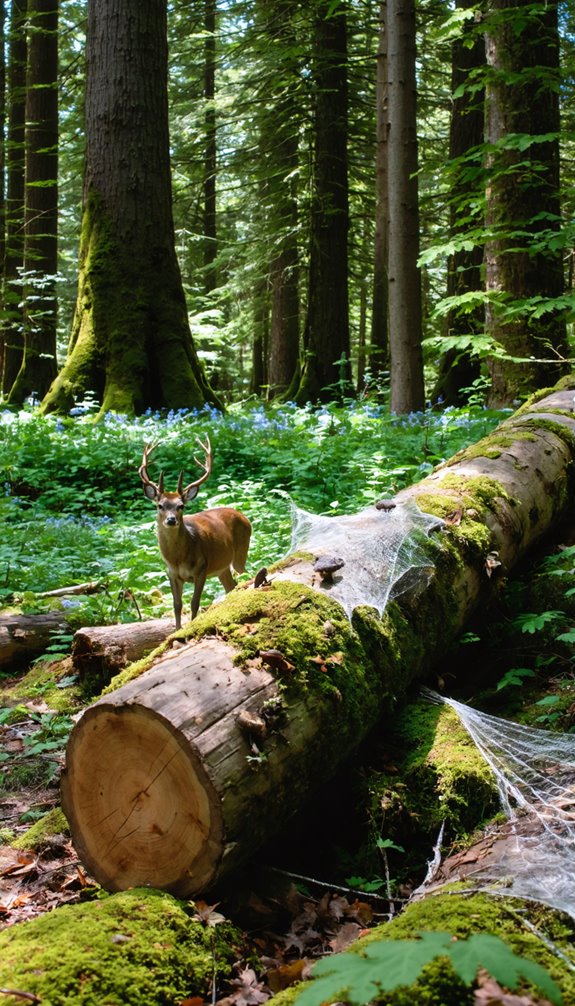
To understand and protect endangered species – and all living organisms – scientists rely on rigorous ecological research methods. When you’re studying ecology, you’ll need to master various data collection techniques, including field surveys, mark-recapture studies, and remote sensing technologies.
You can gather ecological data through direct observation, where you’ll count organisms, measure environmental parameters, and document species interactions. You’ll often use quadrats and transects to sample vegetation, and radio tracking to monitor animal movements. For aquatic ecosystems, you’ll employ nets, traps, and water quality testing equipment.
Modern ecological research also requires you to use sophisticated tools like GPS tracking devices, camera traps, and environmental DNA analysis. You’ll need to understand statistical methods to analyze your data, including population estimates, biodiversity indices, and multivariate analyses.
When conducting ecological research, you must follow strict protocols to guarantee your data is reliable and reproducible. This includes maintaining detailed field notes, using standardized sampling methods, and controlling for environmental variables that might affect your results.
Field Research Techniques
Field research techniques serve as the backbone of ecological studies, giving scientists direct access to valuable data about species and their habitats. You’ll find that these techniques include methods like quadrat sampling, where you’ll place a square frame over an area to count and measure organisms within it. When you’re studying mobile animals, you might use mark-recapture techniques, tagging individuals and later estimating population sizes based on recapture rates.
To track animal movements, you’ll often employ radio telemetry or GPS collars, which let you monitor species’ habitat use and behavior patterns. If you’re studying vegetation, you’ll need to master transect sampling, where you’ll establish a line across a habitat and record all species that intersect it. You’ll also use specialized equipment like soil probes, light meters, and water quality testing kits to measure environmental parameters.
When collecting field data, you’ll need to maintain detailed field notes and use standardized data sheets. You must also follow proper protocols for specimen collection and preservation if you’re gathering physical samples for later analysis in the laboratory.
Data Collection and Analysis
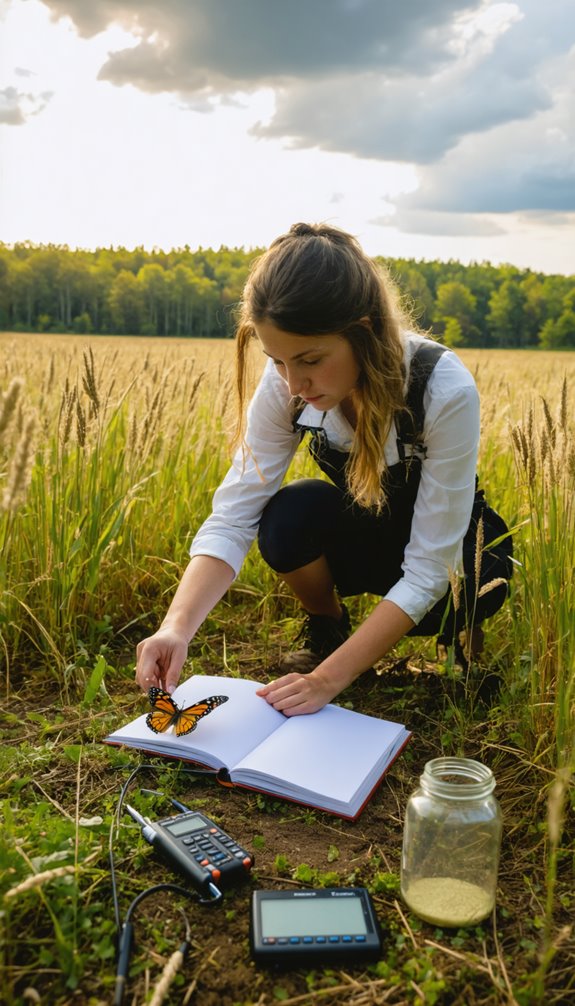
Since ecological research generates vast amounts of raw data, you’ll need systematic methods to collect, organize, and analyze your findings effectively. You’ll want to use standardized data sheets or digital forms to record observations, measurements, and environmental variables consistently. Electronic data loggers and mobile apps can streamline your field data collection while reducing transcription errors.
When you’re organizing data, you’ll find that spreadsheet software like Excel or specialized ecological databases are essential tools. Make sure you’re backing up your data regularly and maintaining clear documentation of your collection methods. You’ll need to establish a consistent naming convention for your files and create separate worksheets for different variables or sampling sites.
For analysis, you’ll typically start with descriptive statistics to understand your data’s basic patterns. You can use statistical software like R or SPSS to perform more complex analyses, including multivariate statistics, regression models, and diversity indices. Don’t forget to create visualizations – graphs and charts will help you identify trends and communicate your findings effectively. Remember to validate your data by checking for outliers and errors before conducting any detailed analysis.
Ecological Modeling
Anyone studying ecology knows that models are essential tools for understanding complex natural systems. You’ll find that ecological models help you predict how populations might change, how species interact, and how ecosystems respond to environmental changes. These models range from simple mathematical equations to sophisticated computer simulations that incorporate multiple variables.
When you’re working with ecological models, you’ll need to reflect on three main types: conceptual models that show relationships through diagrams, mathematical models that use equations to describe ecological processes, and computational models that simulate complex scenarios. You can use these models to test hypotheses without disturbing real ecosystems and to make predictions about future ecological changes.
To create effective models, you’ll want to identify key variables, establish relationships between components, and validate your results against real-world data. It’s essential to remember that models are simplifications of reality, and you must account for their limitations. As you gain experience, you’ll learn to balance model complexity with practical utility, ensuring your predictions remain both accurate and useful for ecological management and research.
Remote Sensing
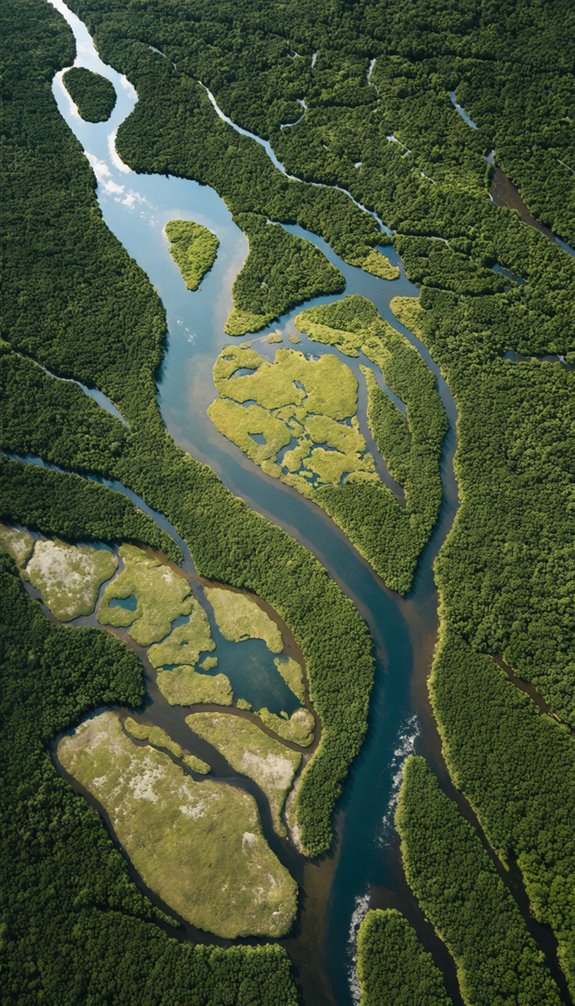
Remote sensing technology has revolutionized the way ecologists study and monitor ecosystems. You’ll find that this technology allows you to collect data about landscapes, vegetation, and wildlife populations without physically being present at the study site. Through satellite imagery, aerial photographs, and drone surveillance, you can track changes in habitat distribution, measure forest cover, and detect environmental disturbances across vast areas.
When you’re using remote sensing tools, you’ll work with different types of electromagnetic radiation data, including visible light, infrared, and radar signals. These various wavelengths help you identify specific features like vegetation health, soil moisture, and land use patterns. You can analyze multispectral images to determine plant productivity, assess drought conditions, or monitor urban development’s impact on natural areas.
Today, you’ll often combine remote sensing with GPS and GIS technologies to create detailed maps and analyze spatial patterns in ecosystems. This integration lets you track wildlife migrations, measure landscape fragmentation, and assess the effects of climate change on biodiversity. You can even use thermal imaging to study animal behavior and monitor population distributions across different habitats.
Geographic Information Systems
Building upon remote sensing data collection, Geographic Information Systems (GIS) provide the tools you’ll need to analyze, visualize, and interpret spatial information about ecosystems. GIS software lets you layer different types of data, from soil composition and elevation to species distributions and climate patterns, creating detailed maps that reveal ecological relationships.
You can use GIS to track changes in habitat boundaries, monitor wildlife movements, and identify areas of conservation concern. The software’s analytical capabilities enable you to perform complex spatial analyses, such as determining habitat connectivity or predicting how climate change might affect species distributions.
When you’re working with GIS, you’ll create digital maps where each feature has associated attributes stored in a database. This means you can query the data to answer specific questions like “Which areas have both high biodiversity and high human population density?” or “Where are the most vulnerable wetland ecosystems?” These capabilities make GIS an essential tool for ecological research, conservation planning, and environmental management decisions. You’ll find it particularly valuable for communicating complex ecological data to stakeholders and the public through clear, visual representations.
Ecological Indicators
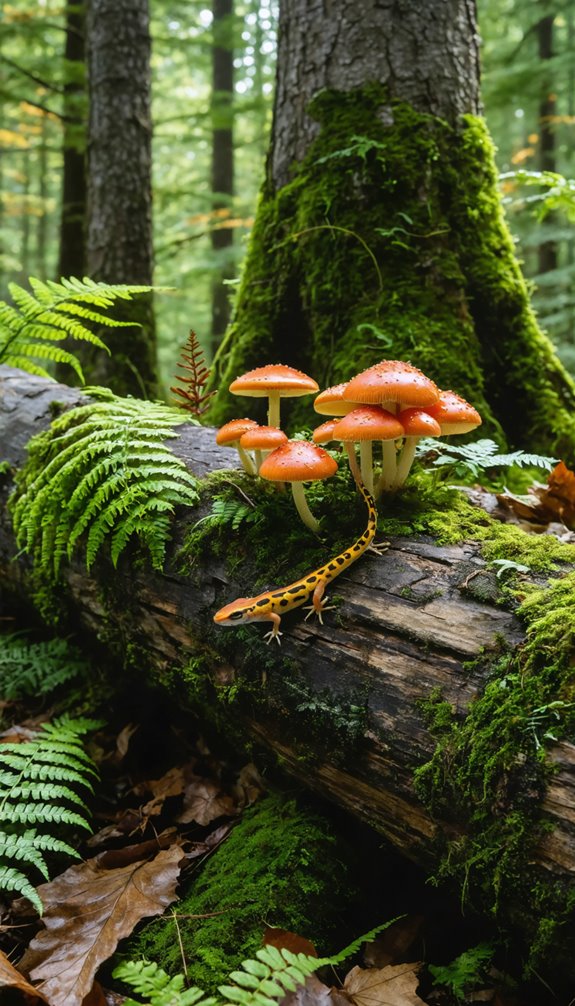
Numerous ecological indicators serve as vital signs that reveal an ecosystem’s health and function. You’ll find these indicators across multiple levels, from individual species to entire landscapes. Key biological indicators include keystone species, whose presence or absence can signal dramatic ecosystem changes, and indicator species that are sensitive to environmental stressors.
When you’re evaluating ecosystem health, you’ll want to monitor both abiotic and biotic indicators. Physical and chemical measurements like water quality, soil pH, and air pollution levels provide essential baseline data. You can track population dynamics, species diversity, and biomass production to understand biological responses to environmental changes.
You’ll notice that some indicators work better than others for specific ecosystems. For instance, lichen species serve as excellent air quality indicators, while macroinvertebrates help you evaluate stream health. Modern ecological monitoring often combines traditional indicators with emerging metrics like genetic diversity and ecosystem services. By regularly measuring these indicators, you can detect environmental changes early, evaluate management strategies, and make informed decisions about conservation efforts.
Monitoring Environmental Change
Environmental monitoring systems create a thorough framework for detecting and tracking ecosystem changes over time. You’ll find these systems employ various tools and methods, from satellite imagery to ground-level sensors, helping scientists understand how environments respond to natural and human-induced pressures. When you’re monitoring environmental change, you’ll need to collect data on multiple parameters, including air quality, water conditions, soil composition, and biodiversity levels.
You can track both short-term fluctuations and long-term trends through continuous data collection. This involves measuring key indicators like temperature variations, precipitation patterns, species populations, and pollutant levels. You’ll need to establish baseline conditions first, then compare subsequent measurements against these initial readings to identify significant changes or patterns.
Modern technology has revolutionized how you can monitor environmental change. You’re now able to use remote sensing, automated weather stations, and environmental DNA sampling to gather data more efficiently. These tools help you detect subtle changes that might otherwise go unnoticed, enabling faster responses to environmental threats and more effective conservation strategies.
Ecosystem Management
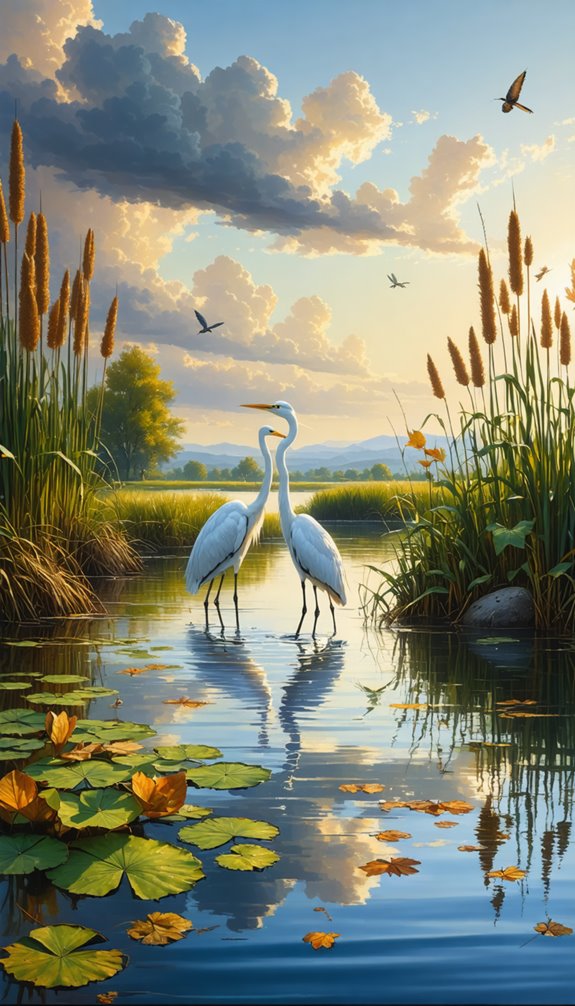
Successful ecosystem management hinges on understanding complex interactions between living organisms and their environment. You’ll need to evaluate multiple factors like biodiversity, resource availability, and human impacts when developing management strategies. By monitoring species populations, habitat quality, and ecosystem services, you can make informed decisions about conservation efforts.
You’ll find that effective ecosystem management requires adaptive approaches. This means you must regularly assess outcomes and adjust your strategies based on new data and changing conditions. You’ll want to implement both short-term actions, like invasive species control, and long-term planning for climate change adaptation.
When you’re managing ecosystems, you’ll need to balance various stakeholder interests. This includes working with local communities, government agencies, and environmental organizations. You’ll discover that successful management often involves combining traditional ecological knowledge with scientific research. Don’t forget to assess economic factors – you must evaluate the costs of conservation measures against the benefits of preserving ecosystem services like water purification, carbon storage, and pollination. Remember that healthy ecosystems provide essential services that support both wildlife and human communities.
Future of ecology
The future of ecology holds both promise and challenge as global ecosystems face unprecedented climate change and habitat destruction. Scientists are increasingly integrating advanced technologies like remote sensing, AI-driven monitoring systems, and environmental DNA analysis to track biodiversity changes with unprecedented precision. Conservation strategies are evolving toward landscape-scale approaches that recognize ecological connectivity across traditional boundaries.
Emerging concepts like rewilding and nature-based solutions offer innovative restoration pathways, while ecological economics works to value ecosystem services in decision-making frameworks. Community-based conservation models that incorporate indigenous knowledge are gaining recognition as effective stewardship approaches. Despite growing extinction threats, collaborative international efforts and technological innovations provide hope that ecological understanding can guide humanity toward more sustainable relationships with natural systems.
Importance of Ecology
Ecology is fundamental to understanding how living organisms interact with each other and their environments. This science helps us identify the complex relationships that sustain biodiversity and ecosystem health. By studying ecological systems, we gain insights into critical issues like climate change, pollution effects, resource management, and species conservation. Ecological knowledge enables sustainable development practices, informs environmental policy, and guides restoration efforts for damaged ecosystems. As humans continue to impact the planet, ecological literacy becomes essential for making informed decisions that balance human needs with environmental protection, ultimately ensuring the survival of countless species, including our own.
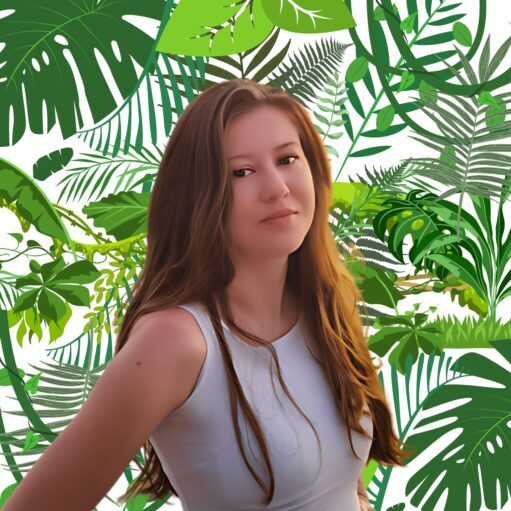
Erzsebet Frey (Eli Frey) is an ecologist and online entrepreneur with a Master of Science in Ecology from the University of Belgrade. Originally from Serbia, she has lived in Sri Lanka since 2017. Eli has worked internationally in countries like Oman, Brazil, Germany, and Sri Lanka. In 2018, she expanded into SEO and blogging, completing courses from UC Davis and Edinburgh. Eli has founded multiple websites focused on biology, ecology, environmental science, sustainable and simple living, and outdoor activities. She enjoys creating nature and simple living videos on YouTube and participates in speleology, diving, and hiking.
- WILDLIFE THEMED T-SHIRTS
Cute Hedgehog Embroidered: Love Wildlife, Protect Nature Wildlife conservation tees
$35.00