Plankton biomass estimation is essential for understanding marine ecosystems and their health. You'll find various methods used, from traditional net sampling to advanced satellite imagery and acoustic sensing. These techniques help scientists measure the abundance of these tiny organisms, which form the base of ocean food webs and play a key role in climate regulation. While challenges like high variability and species diversity exist, new technologies are improving estimation accuracy. Accurate plankton data supports fisheries management, climate change research, and conservation efforts. Dive deeper to explore how these microscopic creatures impact our oceans and global ecosystems.
Why Plankton Biomass Matters
Despite their microscopic size, plankton play an indispensable role in marine ecosystems and the global climate. Understanding plankton biomass is vital for evaluating the health of our oceans and predicting future environmental changes.
You'll find that plankton form the foundation of marine food webs, supporting fish populations and other sea life that humans rely on for food and economic activities.
Plankton biomass serves as an indicator of ocean productivity and can help you gauge the effectiveness of conservation efforts. It's also linked to carbon cycling, as phytoplankton absorb significant amounts of atmospheric CO2 through photosynthesis.
By monitoring plankton biomass, you're able to track changes in ocean chemistry and potential impacts on climate regulation.
Moreover, plankton biomass data is critical for fisheries management, helping to predict fish stock levels and inform sustainable fishing practices. It's also used in climate models to improve predictions of future ocean conditions.
Understanding plankton biomass fluctuations can alert you to ecosystem shifts, potentially signaling broader environmental issues that require attention.
Fundamentally, plankton biomass estimation is a powerful tool for marine science and environmental management.
Traditional Sampling Methods
While modern technology has revolutionized many aspects of marine research, traditional sampling methods still play an essential role in plankton biomass estimation. You'll find that these time-tested techniques provide valuable data that complements newer approaches.
One of the most common methods you'll encounter is net sampling. You'll use plankton nets with specific mesh sizes to collect organisms from various depths. After collection, you'll need to analyze the samples under a microscope to identify and count the different species.
You might also employ water bottles or pumps to collect water samples from specific depths. These methods allow you to capture smaller plankton that might slip through net meshes. Once you've collected the samples, you'll filter them and analyze the contents.
Sediment traps are another traditional method you can use. They collect sinking organic matter, including dead plankton, providing insights into the vertical flux of biomass in the water column.
These methods, while labor-intensive, offer high-resolution data on plankton composition and abundance. They're particularly useful for calibrating and validating newer, automated sampling techniques.
Satellite Imagery Techniques
From high above Earth's surface, satellites provide a powerful tool for estimating plankton biomass across vast oceanic regions. You'll find that these techniques rely on measuring ocean color, which is influenced by the presence of chlorophyll-a in phytoplankton.
Satellites equipped with specialized sensors can detect variations in water color caused by different concentrations of plankton. You'll use algorithms to convert these color measurements into estimates of chlorophyll-a concentration, which serves as a proxy for phytoplankton biomass. This method allows you to cover large areas quickly and repeatedly, providing valuable data on spatial and temporal patterns of plankton distribution.
However, you should be aware of limitations. Satellite imagery can't distinguish between different plankton species or detect subsurface plankton populations. It's also affected by factors like cloud cover and water turbidity. To overcome these challenges, you'll often combine satellite data with in-situ measurements for calibration and validation.
Advanced techniques you might employ include multi-spectral imaging, which uses multiple wavelengths to improve accuracy, and machine learning algorithms to enhance data interpretation. These methods continue to evolve, offering increasingly precise estimates of plankton biomass on a global scale.
Acoustic Sensing Technologies
Acoustic sensing technologies offer a powerful method for estimating plankton biomass beneath the ocean's surface. You'll find that these techniques use sound waves to detect and measure plankton concentrations in the water column. By emitting high-frequency sound pulses and analyzing the echoes, you can gather valuable data on plankton distribution and abundance.
One of the main advantages you'll notice is that acoustic sensing allows for continuous, real-time monitoring over large areas. You can deploy these systems on ships, buoys, or even autonomous underwater vehicles. They're particularly useful for studying zooplankton, as these larger organisms reflect sound waves more effectively than smaller phytoplankton.
When you're using acoustic sensing, you'll need to evaluate factors like frequency selection, calibration, and data interpretation. Different frequencies can target specific size ranges of plankton, so you'll want to choose the right setup for your research goals.
You'll also need to account for potential sources of interference, such as air bubbles or other marine organisms.
Data Analysis and Modeling
Diving into data analysis and modeling is vital when estimating plankton biomass. You'll need to process the raw data collected from various sensing technologies, including acoustic methods. Start by cleaning and pre-processing the data to remove noise and outliers.
Then, you'll apply statistical techniques to identify patterns and trends in plankton distribution and abundance. You'll likely use regression models to establish relationships between environmental variables and plankton biomass.
Machine learning algorithms, such as random forests or neural networks, can help you predict biomass based on multiple input features. Don't forget to validate your models using independent datasets to confirm their accuracy and generalizability.
Time series analysis is essential for understanding temporal variations in plankton populations. You'll want to employ techniques like seasonal decomposition and autoregressive models to capture cyclical patterns and long-term trends.
Spatial analysis tools, including geostatistics and GIS, will help you map plankton distribution across different areas.
Remember to take into account uncertainty in your estimates. You can use Monte Carlo simulations or Bayesian methods to quantify and communicate the confidence intervals of your biomass predictions.
Challenges in Biomass Estimation
Despite advancements in technology and methodology, estimating plankton biomass remains a challenging endeavor. You'll encounter several obstacles when attempting to quantify these microscopic organisms accurately.
One major challenge you'll face is the high spatial and temporal variability of plankton populations. They're constantly in motion, influenced by currents, temperature, and nutrient availability. This makes it difficult to obtain representative samples and extrapolate data to larger areas or time scales.
You'll also struggle with the diversity of plankton species, each with different sizes, shapes, and biochemical compositions. This diversity complicates the conversion of abundance data to biomass estimates. Additionally, you'll need to account for diel vertical migration patterns, where some species move up and down the water column daily.
Sampling methods can introduce biases, too. Net mesh size affects which organisms you capture, potentially underestimating smaller plankton. Preservation techniques can alter organism volume, leading to inaccurate biomass calculations.
Lastly, you'll grapple with the challenge of distinguishing between living and non-living particles in your samples, as detritus can skew biomass estimates if not properly accounted for.
Future Directions and Applications
Looking ahead, you'll find that plankton biomass estimation is poised for significant advancements. Emerging technologies like machine learning and artificial intelligence will revolutionize data analysis, allowing for more accurate and efficient processing of large-scale datasets.
You'll see improved satellite imagery and remote sensing techniques providing near-real-time estimates of plankton distribution across vast ocean areas. In situ sensors and autonomous vehicles will become more sophisticated, offering continuous monitoring capabilities and reducing the need for labor-intensive sampling.
You can expect advancements in genomic and molecular techniques to enhance species identification and biomass estimation at finer scales. These improvements will lead to better understanding of marine ecosystem dynamics and more accurate climate models.
The applications of improved plankton biomass estimation are far-reaching. You'll witness enhanced fisheries management, as plankton serve as an essential food source for many commercial species.
Climate change research will benefit from more precise carbon cycle models. Additionally, you'll see improved predictions of harmful algal blooms and their potential impacts on coastal communities and marine industries.
Ultimately, these advancements will contribute to more effective ocean conservation strategies and sustainable resource management.
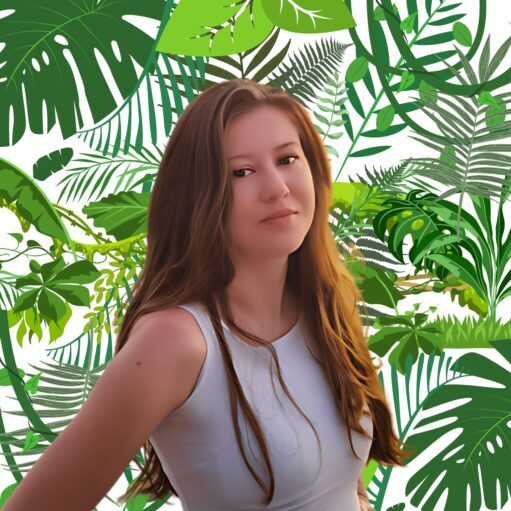
Erzsebet Frey (Eli Frey) is an ecologist and online entrepreneur with a Master of Science in Ecology from the University of Belgrade. Originally from Serbia, she has lived in Sri Lanka since 2017. Eli has worked internationally in countries like Oman, Brazil, Germany, and Sri Lanka. In 2018, she expanded into SEO and blogging, completing courses from UC Davis and Edinburgh. Eli has founded multiple websites focused on biology, ecology, environmental science, sustainable and simple living, and outdoor activities. She enjoys creating nature and simple living videos on YouTube and participates in speleology, diving, and hiking.